Revolutionary materials are transforming modern construction, driving a fundamental shift in how we approach sustainable construction practices and climate resilience. From self-healing concrete that repairs its own cracks using bacteria to transparent aluminum composites offering unprecedented strength-to-weight ratios, these innovations are redefining structural possibilities while dramatically reducing environmental impact.
Advanced engineered wood products, including mass timber and cross-laminated timber (CLT), now enable the construction of high-rise buildings while sequestering carbon and reducing embodied energy by up to 45% compared to traditional materials. Similarly, graphene-enhanced building components deliver exceptional strength and conductivity while requiring minimal raw materials, representing a crucial advancement in resource-efficient construction.
These breakthrough materials aren’t just theoretical possibilities—they’re being implemented in projects worldwide, demonstrating superior performance metrics and long-term cost benefits. As climate challenges intensify, these innovations offer construction professionals practical solutions that combine structural integrity with environmental responsibility, marking a pivotal evolution in building technology.
Self-Healing Materials: The Future of Sustainable Infrastructure
Bacterial Concrete Technology
Bacterial concrete technology represents a groundbreaking advancement in developing climate-resilient infrastructure, utilizing microorganisms to create self-healing properties in concrete structures. This innovative material incorporates specific strains of bacteria, typically Bacillus subtilis or Bacillus sphaericus, along with calcium-based nutrients into the concrete mixture during production.
When cracks develop in the concrete, water exposure activates the dormant bacteria. These microorganisms then metabolize the calcium-based nutrients, producing limestone (calcium carbonate) that effectively seals the cracks. This autonomous healing process can repair fissures up to 0.8mm wide within 28 days under optimal conditions.
Field trials have demonstrated remarkable durability improvements, with bacterial concrete structures showing 20-30% higher compression strength compared to conventional concrete. The technology has proven particularly effective in water-retaining structures, underground constructions, and marine environments where water infiltration poses significant risks.
While implementation costs currently exceed traditional concrete by 10-15%, the extended service life and reduced maintenance requirements offer compelling long-term economic benefits. Leading construction firms report maintenance cost reductions of up to 50% over the structure’s lifetime, making bacterial concrete an increasingly attractive option for sustainable infrastructure development.
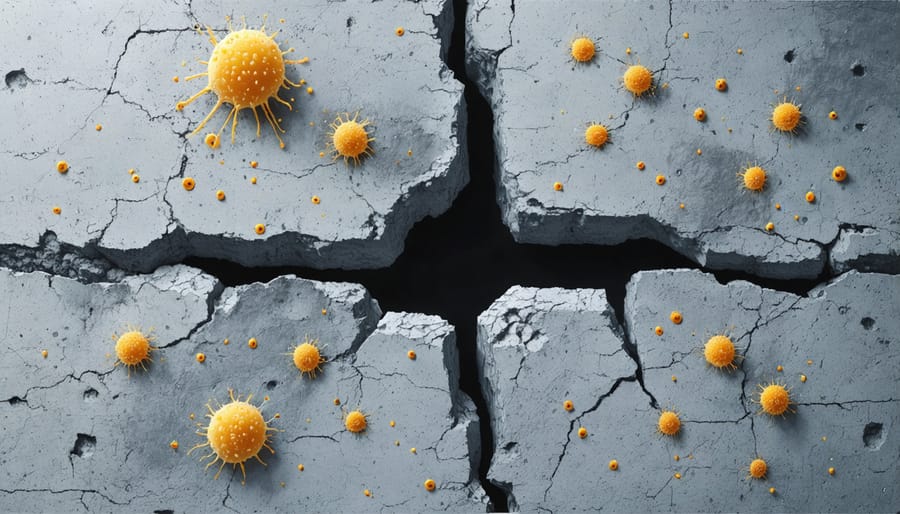
Shape Memory Materials
Shape memory materials (SMMs) represent a groundbreaking advancement in construction technology, offering the remarkable ability to return to their original form after experiencing deformation. These smart materials, primarily shape memory alloys (SMAs) and shape memory polymers (SMPs), respond to specific environmental triggers such as temperature changes or mechanical stress.
Nickel-titanium (NiTi) alloys, commonly known as Nitinol, lead the field in construction applications. These materials can absorb significant deformation energy and recover their initial shape through either heating or stress removal, making them invaluable for seismic protection systems and structural reinforcement.
Recent implementations include self-healing bridge joints that automatically close gaps caused by thermal expansion and smart facades that adapt to environmental conditions. Engineers have successfully utilized SMMs in structural damping systems, where they help buildings absorb and dissipate energy during earthquakes.
The cost-effectiveness of SMMs continues to improve as manufacturing processes advance. While initial investment remains higher than traditional materials, their long-term benefits in reducing maintenance requirements and enhancing structural resilience often justify the expense. Research indicates that incorporating SMMs into critical structural components can extend a building’s service life by up to 30% while significantly improving its ability to withstand extreme events.
Advanced Composites for Extreme Weather Resistance
Carbon Fiber Reinforced Polymers
Carbon Fiber Reinforced Polymers (CFRP) represent one of the most significant advancements in structural reinforcement technology, offering exceptional strength-to-weight ratios that far exceed traditional materials. These composites, consisting of carbon fiber embedded in a polymer matrix, deliver tensile strengths up to ten times greater than steel while weighing just one-quarter as much.
In modern construction, CFRP applications have become increasingly diverse, particularly in projects requiring innovative resilience solutions. The material excels in structural retrofitting, seismic strengthening, and bridge rehabilitation, where its corrosion resistance and durability provide crucial advantages over conventional reinforcement methods.
Recent technological advances have improved CFRP’s cost-effectiveness and installation efficiency. Pre-manufactured CFRP sheets and strips can now be rapidly applied using specialized adhesives, reducing construction time and labor costs. The material’s flexibility allows for custom solutions in complex architectural designs, while its non-intrusive nature makes it ideal for historical building preservation.
Despite higher initial costs compared to traditional materials, CFRP’s extended service life and minimal maintenance requirements often result in lower life-cycle costs. Industry data suggests that CFRP-reinforced structures can maintain their structural integrity for over 50 years with proper installation and protection from UV exposure.
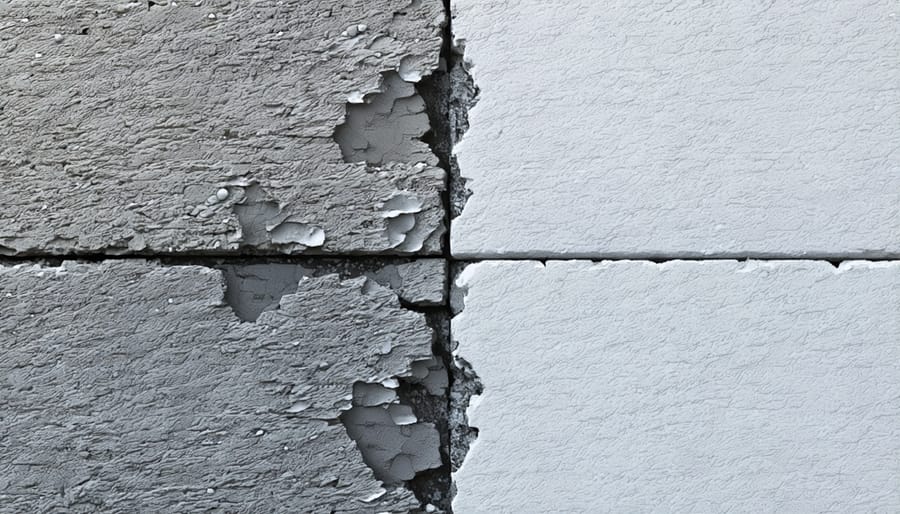
Nano-Enhanced Materials
Nanotechnology has revolutionized construction materials by enhancing their properties at the molecular level. Through the incorporation of nanoparticles and nanostructures, traditional building materials exhibit significantly improved strength, durability, and functionality. Concrete, the most widely used construction material globally, benefits particularly from nano-enhancement through the addition of materials like nano-silica and carbon nanotubes, resulting in compressive strength increases of up to 40%.
These nano-enhanced materials demonstrate remarkable self-cleaning properties through the incorporation of titanium dioxide nanoparticles, which break down organic pollutants when exposed to sunlight. Additionally, nanomaterials can create surfaces with superior water and stain resistance, reducing maintenance requirements and extending material lifespans.
Smart sensing capabilities are another breakthrough, with embedded nanosensors enabling real-time monitoring of structural integrity and environmental conditions. Recent developments include self-healing materials that utilize nanoencapsulated healing agents to automatically repair micro-cracks before they develop into significant structural issues.
Research from the Massachusetts Institute of Technology demonstrates that nano-modified materials can reduce carbon emissions during production by up to 25% while improving thermal insulation properties. However, implementation challenges remain, including higher initial costs and the need for specialized application techniques. Industry experts predict that as manufacturing processes become more refined, the cost-benefit ratio will continue to improve, making nano-enhanced materials increasingly accessible for mainstream construction projects.
Smart Materials for Environmental Adaptation
Phase Change Materials
Phase Change Materials (PCMs) represent a significant advancement in passive temperature regulation for buildings, offering an innovative solution for energy-efficient climate control. These materials function by absorbing or releasing thermal energy during phase transitions, typically between solid and liquid states, at specific temperature thresholds.
Common PCMs in construction include paraffin compounds, salt hydrates, and bio-based materials, each engineered to operate within precise temperature ranges. When integrated into building materials such as wallboards, concrete, or insulation systems, PCMs can absorb excess heat during peak daytime temperatures and release it when temperatures drop, creating a natural thermal buffering effect.
Research indicates that properly implemented PCM systems can reduce HVAC energy consumption by 20-30% in commercial buildings. For example, a recent office development in Frankfurt demonstrated annual cooling load reductions of 25% after incorporating PCM-enhanced drywall panels.
Implementation methods include microencapsulation, where tiny PCM particles are embedded within construction materials, and macro-encapsulation, where larger quantities are contained within discrete modules. The selection of specific PCM types depends on factors such as desired operating temperature, building usage patterns, and local climate conditions.
While initial costs remain higher than traditional materials, the long-term energy savings and improved occupant comfort make PCMs increasingly attractive for sustainable construction projects. Industry experts project continued advancement in PCM technology, with new compounds offering enhanced performance and durability.
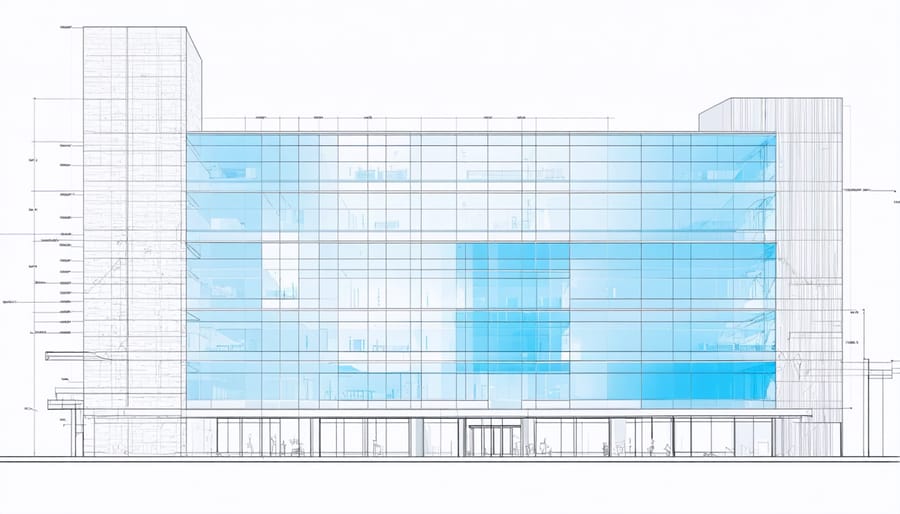
Photochromic and Thermochromic Systems
Photochromic and thermochromic systems represent a significant advancement in adaptive building materials, offering dynamic responses to environmental conditions. These smart materials automatically adjust their properties based on external stimuli, enhancing both energy efficiency and occupant comfort.
Photochromic materials react to light intensity by changing their optical properties. When exposed to UV radiation, these materials undergo reversible color changes, effectively controlling solar heat gain and glare. Modern photochromic glazing systems can reduce cooling loads by up to 20% compared to conventional windows, making them particularly valuable in commercial buildings with large glass facades.
Thermochromic systems, conversely, respond to temperature fluctuations. These materials can alter their transparency or color based on thermal conditions, providing passive temperature regulation. For instance, thermochromic concrete aggregates can reflect more sunlight during hot weather, while thermochromic window films adjust their tint to optimize indoor temperatures throughout the day.
Recent developments have integrated both technologies into hybrid systems. These advanced materials combine light and temperature sensitivity, offering more sophisticated environmental adaptation. A notable example is the development of smart facades that incorporate both properties, providing optimal performance across varying climate conditions.
Implementation costs remain a consideration, but the long-term energy savings and enhanced occupant comfort often justify the investment. As manufacturing processes improve and market demand increases, these adaptive materials are becoming increasingly accessible to construction professionals.
Implementation and Cost Considerations
Economic Viability
The economic viability of innovative construction materials presents a complex cost-benefit equation that industry professionals must carefully evaluate. While initial costs typically exceed traditional options by 15-30%, long-term financial benefits often justify the investment. For instance, advanced composite materials, though commanding a premium of approximately $75-100 per square foot over conventional materials, demonstrate superior durability and reduced maintenance requirements, potentially saving 40% in lifecycle costs over 30 years.
Energy-efficient materials like aerogel insulation and smart glass, while initially expensive, can reduce building operating costs by 25-35% annually. This translates to significant returns on investment, typically achieved within 5-7 years of implementation. Companies revolutionizing sustainable building through innovative materials have documented cost recovery periods shortening as manufacturing scales increase and technology matures.
Recent market analysis indicates that buildings incorporating these materials command 7-10% higher resale values and attract premium tenants, contributing to enhanced revenue streams. Insurance companies increasingly offer reduced premiums for structures utilizing climate-resilient materials, recognizing their superior performance during extreme weather events. Furthermore, government incentives and green building certifications can offset initial costs by 10-20%, making the transition to innovative materials more financially attractive for developers and property owners.
However, successful implementation requires careful consideration of regional availability, installation expertise, and maintenance requirements to optimize the economic benefits while ensuring long-term performance.
Integration Challenges and Solutions
While innovative construction materials offer remarkable benefits, their integration into existing construction practices presents several challenges that require strategic solutions. Material compatibility remains a primary concern, particularly when combining new materials with traditional ones. Construction teams must carefully evaluate potential chemical reactions and structural interactions to ensure long-term stability.
Quality control and standardization pose another significant challenge. Many innovative materials lack established industry standards, making it difficult to ensure consistent performance across projects. To address this, leading construction firms are developing comprehensive testing protocols and working with certification bodies to establish reliable benchmarks.
Training and workforce adaptation represent crucial implementation hurdles. Construction teams need specialized knowledge to handle new materials effectively. Progressive companies are addressing this through comprehensive training programs and partnerships with material manufacturers to provide hands-on experience and technical support.
Supply chain considerations also impact successful integration. Limited availability and inconsistent supply of innovative materials can affect project timelines and costs. Solutions include early procurement planning, maintaining relationships with multiple suppliers, and considering local manufacturing options where feasible.
Cost management remains a critical factor. While innovative materials may have higher upfront costs, successful implementation requires demonstrating long-term value through reduced maintenance, enhanced performance, and improved sustainability metrics. Project managers are developing detailed cost-benefit analyses that account for the complete lifecycle of these materials, helping stakeholders understand their true economic value.
These challenges, while significant, can be effectively managed through careful planning, proper training, and strategic implementation approaches.
The emergence of innovative construction materials marks a pivotal shift in how we approach climate-resilient building practices. These advanced materials not only address immediate construction challenges but also lay the groundwork for a more sustainable and resilient built environment. Through the integration of self-healing concrete, transparent wood composites, and carbon-negative materials, the construction industry is positioned to significantly reduce its environmental impact while enhancing structural durability.
The economic implications of these innovations extend beyond initial material costs. Long-term benefits include reduced maintenance requirements, extended building lifespans, and enhanced resilience to extreme weather events. Industry leaders report up to 40% reduction in lifecycle costs when implementing these advanced materials in their projects.
Moreover, the adaptability of these materials to various climate conditions positions them as crucial elements in future-proofing construction projects. Their ability to respond dynamically to environmental stresses while maintaining structural integrity represents a significant advancement in building technology.
Looking ahead, the widespread adoption of these innovative materials will likely accelerate as manufacturing processes become more efficient and cost-effective. This evolution in construction materials is not just about meeting current climate challenges; it’s about reimagining the very foundation of how we build for future generations. As regulatory frameworks increasingly emphasize sustainability and resilience, these materials will become standard elements in construction practices, driving the industry toward a more sustainable and climate-resilient future.