The power grid stands at the cusp of its most significant transformation since the early 20th century. Smart grid systems, revolutionizing how we generate, distribute, and consume electricity, represent a fundamental shift from traditional one-way power distribution to an intelligent, interactive network. Understanding the fundamental principles of smart grids has become crucial for construction and energy professionals navigating the evolving landscape of sustainable infrastructure.
This advanced power infrastructure integrates sophisticated digital communications, automated controls, and real-time monitoring capabilities, enabling unprecedented levels of grid reliability and efficiency. By incorporating artificial intelligence, IoT sensors, and advanced metering systems, smart grids optimize power flow, reduce outages, and facilitate the seamless integration of renewable energy sources into existing networks.
For construction professionals and project stakeholders, smart grid technology represents not just an upgrade to existing systems, but a complete reimagining of how buildings interact with power infrastructure. This transformation demands new approaches to project planning, implementation, and maintenance, fundamentally changing how we design and construct energy-efficient buildings for the future.
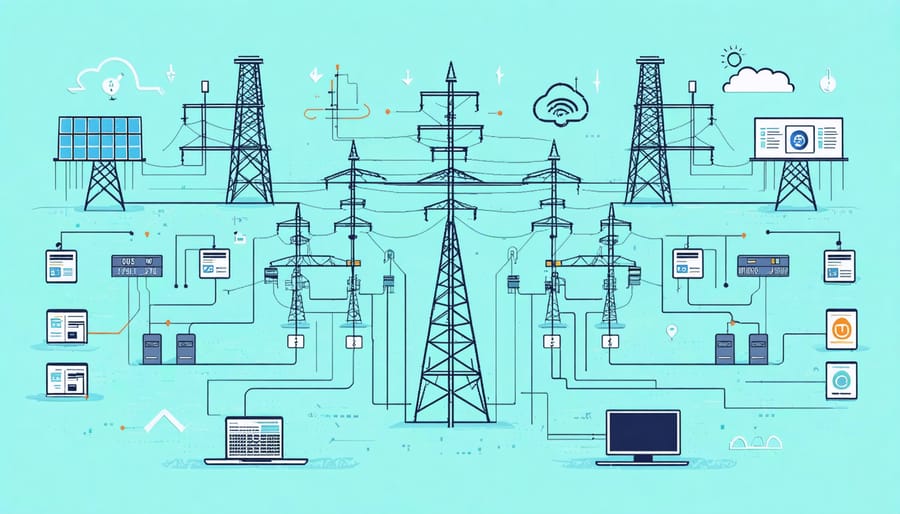
The Architecture of Modern Smart Grid Systems
Advanced Metering Infrastructure (AMI)
Advanced Metering Infrastructure (AMI) represents a cornerstone of modern smart grid systems, enabling two-way communication between utilities and consumers through sophisticated smart meters and data collection networks. These smart meters continuously collect and transmit real-time energy consumption data, replacing traditional manual meter reading with automated, digital measurements.
The infrastructure consists of three primary components: smart meters at customer locations, a secure communications network, and data management systems at the utility level. Smart meters record consumption data at intervals typically ranging from 15 minutes to one hour, providing granular insights into energy usage patterns. They also detect power quality issues, outages, and unauthorized tampering attempts.
The communications network utilizes various technologies, including radio frequency (RF) mesh networks, cellular systems, or power line communications (PLC), to transmit data securely between meters and utility control centers. This network architecture ensures reliable data transmission while maintaining cybersecurity protocols to protect sensitive information.
At the utility level, Meter Data Management Systems (MDMS) process and analyze the collected data, enabling utilities to optimize grid operations, implement dynamic pricing strategies, and provide customers with detailed consumption information through web portals or mobile applications. The system also supports automated billing, remote connection/disconnection capabilities, and enhanced outage management.
Recent implementations of AMI systems have demonstrated significant operational improvements, with utilities reporting up to 95% reduction in meter reading costs and substantial improvements in outage response times.
Grid Automation and Control Systems
Grid automation and control systems represent the central nervous system of modern smart grids, integrating advanced monitoring, control, and communication technologies to enhance power distribution efficiency. These sophisticated smart energy management systems utilize real-time data analytics and automated decision-making processes to optimize grid performance and reliability.
At the core of these systems are Advanced Distribution Management Systems (ADMS), which combine SCADA functionality with distribution management and outage management capabilities. These systems enable utilities to monitor grid conditions, detect faults, and implement automatic restoration protocols without human intervention. Real-time monitoring through smart sensors and meters provides continuous feedback on grid health, power quality, and consumption patterns.
Automated switching and self-healing capabilities allow the grid to isolate faults and reroute power automatically, significantly reducing outage duration and impact. Advanced algorithms process vast amounts of data to predict potential issues before they occur, enabling preventive maintenance and reducing system downtime.
The integration of distributed energy resources (DERs) is managed through sophisticated control systems that balance supply and demand in real-time. These systems coordinate multiple power sources, including renewable energy installations, storage systems, and traditional generation, ensuring stable power delivery while optimizing resource utilization and reducing operational costs.
Real-Time Grid Intelligence and Management
Demand Response Management
Demand Response Management (DRM) represents a critical component of modern smart grid infrastructure, enabling utilities and facility managers to maintain grid stability through intelligent load balancing. By leveraging advanced distributed energy management systems, operators can effectively respond to peak demand periods while minimizing disruption to end users.
The system operates through a combination of automated controls and user participation protocols. During high-demand periods, the smart grid can automatically adjust power consumption across connected facilities, prioritizing critical operations while temporarily reducing non-essential loads. This dynamic load management capability typically achieves 15-20% reduction in peak demand, resulting in significant cost savings and improved grid reliability.
Key strategies implemented in modern DRM systems include:
– Time-of-use pricing mechanisms that incentivize off-peak consumption
– Automated load shedding protocols for non-critical systems
– Real-time monitoring and predictive analytics for demand forecasting
– Integration with building automation systems for seamless response
– Direct load control programs for large commercial consumers
Recent case studies demonstrate that buildings equipped with advanced DRM capabilities achieve average energy cost reductions of 12-18% annually. Furthermore, these systems provide essential grid support during extreme weather events or unexpected supply disruptions, maintaining power stability across the network.
For construction professionals, implementing DRM-ready infrastructure during new construction or renovations has become increasingly important. This includes installing smart meters, automated switching systems, and compatible building management systems that can participate in demand response programs while maintaining occupant comfort and operational efficiency.
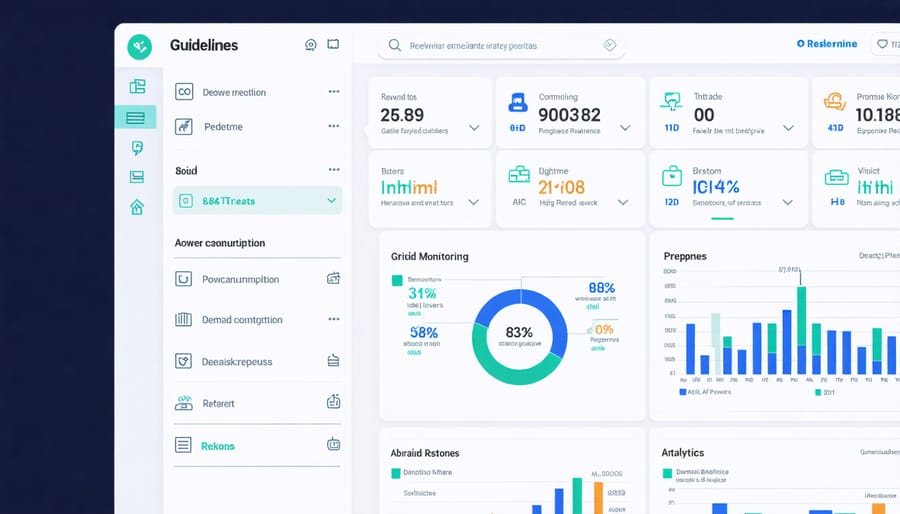
Predictive Analytics and AI Integration
Predictive analytics and artificial intelligence have revolutionized smart grid operations, enabling unprecedented levels of efficiency and reliability in power distribution networks. These advanced technologies analyze vast amounts of real-time data from sensors, meters, and grid infrastructure to forecast demand patterns, identify potential failures, and optimize resource allocation.
Machine learning algorithms continuously process historical and current grid performance data to predict maintenance requirements before equipment failures occur. This proactive approach has reduced unexpected outages by up to 50% in implemented systems, while cutting maintenance costs by 20-30%. AI-powered load forecasting helps utilities anticipate demand spikes and adjust power distribution accordingly, ensuring optimal grid stability during peak usage periods.
Grid operators now employ sophisticated neural networks to analyze weather patterns, consumption trends, and equipment health indicators. These systems can detect anomalies in power quality, identify cyber security threats, and automatically implement corrective measures. For example, smart transformers equipped with AI capabilities can self-regulate voltage levels and redistribute power flows to prevent overloading.
The integration of AI has also enhanced renewable energy integration by better managing intermittent power sources. Predictive models forecast solar and wind power generation with up to 97% accuracy, allowing for more efficient energy storage and distribution strategies. This capability has proven crucial for maintaining grid stability as renewable energy adoption increases.
Recent implementations have demonstrated that AI-driven smart grids can achieve a 15% reduction in power losses and a 30% improvement in asset utilization. As these technologies continue to evolve, they’re becoming increasingly essential for modern power infrastructure management, offering both operational efficiency and enhanced grid resilience.
Integration of Renewable Energy Sources
Grid Storage Solutions
Grid storage solutions represent a critical component in modern smart grid infrastructure, serving as the backbone for reliable power distribution and renewable energy integration. Energy storage systems (ESS) primarily utilize advanced battery technologies, including lithium-ion arrays, flow batteries, and emerging solid-state solutions, to maintain grid stability and manage peak demand fluctuations.
Large-scale battery installations, ranging from 10MW to 100MW capacity, provide essential services such as frequency regulation, voltage support, and load shifting. These systems can respond to demand changes within milliseconds, offering superior grid stability compared to traditional power plants. For example, the Hornsdale Power Reserve in South Australia demonstrates how a 150MW battery installation can effectively support grid reliability while enabling greater renewable energy integration.
Thermal energy storage systems, including molten salt and phase-change materials, offer complementary storage solutions, particularly for solar thermal plants. These systems can maintain power output during cloud cover or after sunset, ensuring consistent energy delivery to the grid.
Mechanical storage solutions, such as pumped hydro storage and flywheel systems, continue to play vital roles in grid stability. While pumped hydro remains the most widely deployed storage technology globally, modern flywheel installations provide rapid-response frequency regulation services in urban environments where space is limited.
The integration of distributed storage systems at both utility and building levels creates a more resilient grid architecture, enabling bidirectional power flow and improved demand response capabilities.
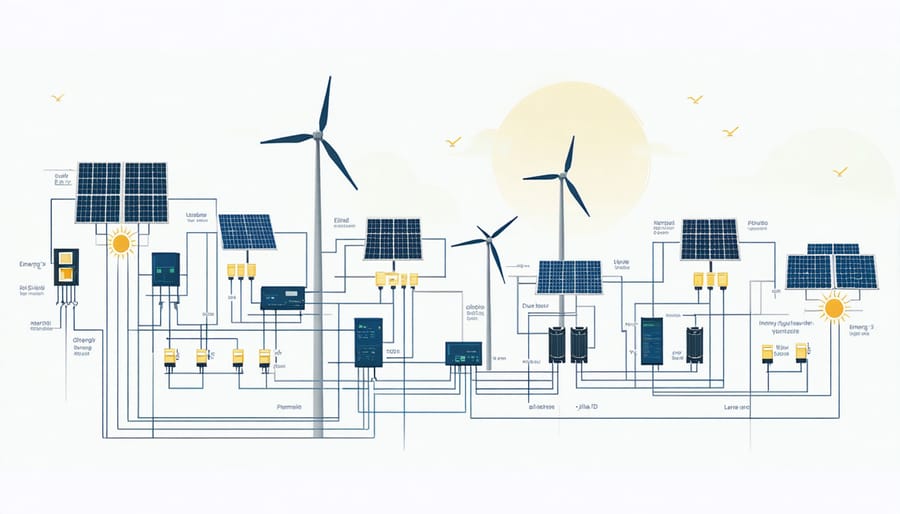
Microgrid Implementation
Microgrids represent a critical advancement in smart grid implementation, offering localized power distribution networks that can operate either independently or in conjunction with the main grid. These systems typically incorporate distributed energy resources (DERs), including solar panels, wind turbines, and energy storage systems, creating a resilient and flexible power infrastructure.
The architecture of a microgrid consists of three primary components: power generation sources, energy storage systems, and sophisticated control systems. Advanced metering infrastructure (AMI) and power electronics enable seamless switching between grid-connected and island modes, ensuring continuous power supply during main grid disruptions.
Implementation benefits are particularly compelling for commercial and industrial facilities. Studies show that microgrids can reduce energy costs by 15-25% through optimized load management and peak shaving capabilities. Healthcare facilities, data centers, and manufacturing plants have reported significant improvements in power reliability, with some achieving 99.999% uptime.
Recent case studies demonstrate successful microgrid deployments across various sectors. For instance, a manufacturing facility in Detroit implemented a microgrid system that integrated 2MW of solar capacity with 1MWh of battery storage, resulting in a 30% reduction in energy costs and enhanced operational resilience during grid outages.
The integration of microgrids within smart grid systems represents a fundamental shift toward more resilient, efficient, and sustainable power distribution networks, offering organizations greater control over their energy resources while supporting grid stability.
Future-Proofing Grid Infrastructure
As we look toward the horizon of smart grid development, several emerging trends are reshaping how we approach grid infrastructure. The integration of artificial intelligence and machine learning algorithms is enabling predictive maintenance capabilities that can anticipate and prevent system failures before they occur. These advanced analytics systems process vast amounts of real-time data from grid sensors, transforming reactive maintenance into proactive grid management.
Energy storage technologies are evolving rapidly, with next-generation batteries and innovative storage solutions becoming increasingly vital for grid stability. Grid-scale battery installations are expanding, while new technologies like flow batteries and hydrogen storage systems are showing promise for longer-duration energy storage needs. These developments are crucial for managing the intermittent nature of renewable energy sources and ensuring consistent power delivery.
The concept of grid resilience is taking center stage, particularly in response to climate change challenges. Modern smart grids are being designed with self-healing capabilities, allowing them to automatically isolate faults and reroute power flow to minimize disruptions. This includes implementing advanced protection schemes and developing microgrids that can operate independently during emergencies.
Cybersecurity measures are becoming more sophisticated to protect against evolving threats. The industry is moving toward zero-trust architecture and implementing blockchain technology for secure transactions and data management. These security frameworks are essential as grid systems become more interconnected and digitalized.
Edge computing is emerging as a critical component of future grid infrastructure, enabling faster processing of data closer to its source. This distributed computing approach reduces latency in grid operations and supports real-time decision-making capabilities.
Looking ahead, the integration of electric vehicles (EVs) will significantly impact grid design. Vehicle-to-grid (V2G) technology is expected to transform EVs from mere power consumers into mobile energy storage units that can support grid stability during peak demand periods.
These developments are driving the evolution of regulatory frameworks and industry standards. Grid operators and utilities are working closely with technology providers to establish protocols that ensure interoperability and security while maintaining flexibility for future innovations. The key to success lies in creating adaptable infrastructure that can accommodate emerging technologies while maintaining reliability and efficiency.
Smart grid systems represent a pivotal advancement in power distribution infrastructure, fundamentally transforming how we generate, distribute, and consume electricity. By integrating advanced digital communications, real-time monitoring, and automated control systems, smart grids have become essential for meeting the evolving demands of modern energy consumption while enhancing grid reliability and efficiency.
The implementation of smart grid technology offers substantial benefits for construction projects and facility operations, including reduced energy costs, improved power quality, and enhanced sustainability. These systems enable better integration of renewable energy sources, support the growing adoption of electric vehicles, and provide crucial data insights for informed decision-making in facility management.
Looking ahead, smart grid systems will continue to play a crucial role in shaping the future of power distribution. Their ability to support bidirectional power flow, self-healing capabilities, and demand response mechanisms positions them as a cornerstone of sustainable urban development. For construction professionals and facility managers, understanding and leveraging smart grid capabilities has become fundamental to delivering energy-efficient, future-ready projects that meet both regulatory requirements and client expectations.