Infrastructure resilience stands at the forefront of modern engineering challenges, where the convergence of climate change, cyber threats, and aging systems demands unprecedented attention. As critical networks face escalating risks from natural disasters, technological disruptions, and security breaches, the imperative to build robust, adaptable infrastructure has never been more urgent.
Recent data reveals that infrastructure failures cost global economies over $300 billion annually, with 40% of these incidents stemming from preventable vulnerabilities. Forward-thinking organizations now implement comprehensive resilience strategies that integrate advanced materials, smart monitoring systems, and adaptive management protocols to ensure continuous operation under stress.
This evolution in infrastructure design and maintenance represents a fundamental shift from traditional reliability-centered approaches to dynamic resilience frameworks. Modern infrastructure must not merely withstand challenges but adapt and recover swiftly from disruptions. By incorporating predictive analytics, distributed systems, and regenerative design principles, organizations can develop infrastructure networks that maintain essential services while rapidly responding to emerging threats.
The intersection of digital transformation and physical infrastructure creates new opportunities for enhancing system resilience through real-time monitoring, automated response mechanisms, and integrated risk management platforms. These innovations, coupled with stringent regulatory standards and industry best practices, form the foundation of next-generation infrastructure that safeguards communities and economic assets against an increasingly unpredictable future.
This paradigm shift in infrastructure resilience demands a coordinated effort among engineers, policymakers, and stakeholders to implement robust solutions that protect critical systems while enabling sustainable growth and adaptation to emerging challenges.
The Evolution of Energy Infrastructure Vulnerabilities
Climate Change Impact Assessment
Climate change continues to pose unprecedented challenges to infrastructure systems worldwide, with extreme weather events increasing in both frequency and intensity. Recent studies indicate that infrastructure damage from climate-related incidents has risen by 68% over the past decade, particularly affecting transportation networks, energy systems, and water management facilities.
Severe weather events, including hurricanes, floods, and prolonged heat waves, create multiple stress points in infrastructure systems. Heat waves can cause rail tracks to buckle and road surfaces to deteriorate, while increased flooding threatens underground utilities and foundation stability. In coastal regions, rising sea levels combined with storm surges pose significant risks to critical facilities and transportation networks.
The financial impact of these climate-related infrastructure failures is substantial. According to industry analysis, the global cost of climate-induced infrastructure damage reached $300 billion in 2022, with projections indicating this figure could triple by 2050 without adequate adaptation measures.
Engineering assessments reveal that traditional infrastructure design standards, based on historical weather patterns, are becoming increasingly inadequate. Modern infrastructure must account for future climate scenarios, incorporating greater safety margins and adaptive capacity. This includes designing structures to withstand higher wind loads, implementing improved drainage systems, and utilizing materials that can better withstand temperature extremes.
Case studies from major urban centers demonstrate that proactive climate adaptation strategies in infrastructure design can reduce long-term maintenance costs by up to 40% while significantly improving system reliability during extreme weather events.
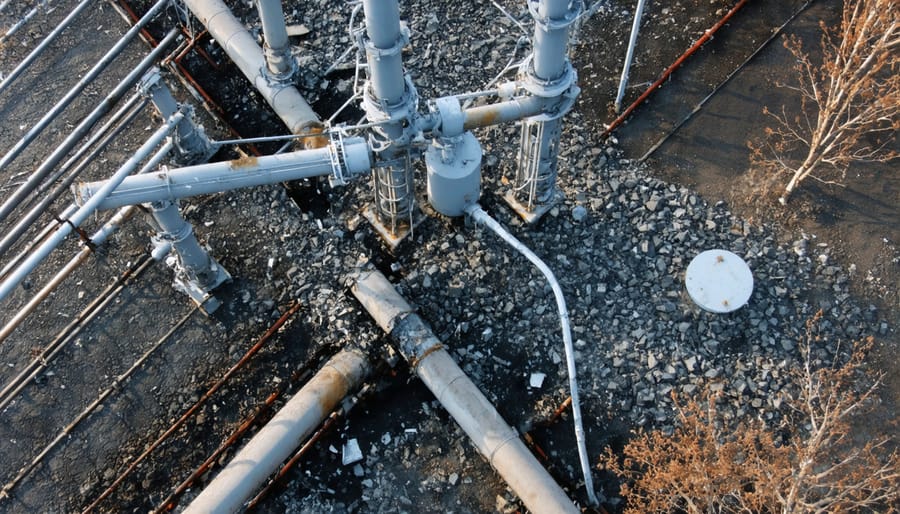
Cybersecurity Considerations
The increasing digitalization of energy infrastructure has introduced new vulnerabilities that require robust cybersecurity measures. Smart grids, automated control systems, and IoT devices, while enhancing operational efficiency, have expanded the attack surface for cyber threats. Recent incidents have shown that sophisticated attackers can potentially disrupt power distribution, manipulate industrial control systems, and compromise critical infrastructure components.
To maintain infrastructure resilience, organizations must implement multi-layered security approaches. This includes network segmentation, regular security audits, and advanced threat detection systems. The adoption of encryption protocols for data transmission, secure authentication mechanisms, and continuous monitoring of network traffic are essential components of a comprehensive cybersecurity strategy.
Particularly critical is the protection of SCADA (Supervisory Control and Data Acquisition) systems, which form the backbone of modern energy infrastructure. These systems require specialized security measures, including air-gapped networks where appropriate, and rigorous access control protocols.
Industry standards such as IEC 62443 and NIST frameworks provide valuable guidelines for securing industrial control systems. Organizations should also establish incident response plans and conduct regular cybersecurity drills to ensure preparedness for potential attacks.
The human element remains crucial, with staff training and security awareness programs playing vital roles in preventing social engineering attacks and maintaining overall system integrity. Regular updates to security protocols and technology systems help address emerging threats and vulnerabilities.
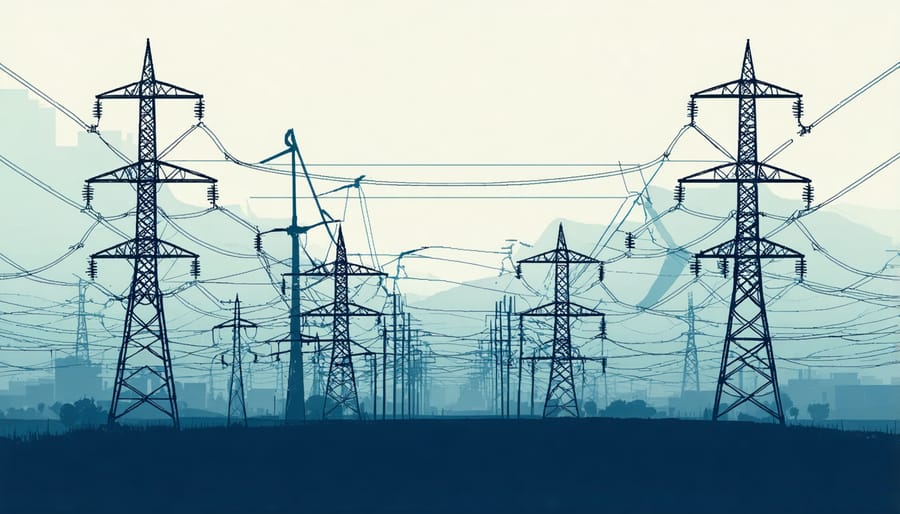
Advanced Resilience Technologies and Strategies
Smart Grid Integration
The integration of smart grid systems represents a transformative approach to infrastructure resilience, combining advanced automation with real-time monitoring capabilities. These sophisticated networks utilize artificial intelligence and machine learning algorithms to analyze vast amounts of operational data, enabling predictive maintenance and rapid response to potential system failures.
Central to smart grid functionality is the implementation of Advanced Metering Infrastructure (AMI) and Supervisory Control and Data Acquisition (SCADA) systems. These technologies provide operators with granular insights into grid performance, power quality, and consumption patterns. Real-time monitoring enables immediate detection of anomalies, from voltage fluctuations to equipment malfunctions, allowing for proactive intervention before issues escalate.
The integration of automated fault detection and self-healing capabilities significantly enhances grid resilience. When disruptions occur, smart systems can automatically isolate affected areas and reroute power through alternative pathways, minimizing service interruptions. This dynamic response capability is particularly crucial during extreme weather events or cyber threats.
Recent case studies demonstrate the effectiveness of smart grid integration. For instance, during Hurricane Sandy, utilities with advanced grid systems reduced outage duration by up to 55% compared to traditional infrastructure. These systems also optimize resource allocation by automatically adjusting power distribution based on demand patterns and available renewable energy sources.
To maximize the benefits of smart grid integration, organizations must invest in robust cybersecurity measures and ensure system interoperability. Regular testing and updates of automated systems, combined with comprehensive staff training, are essential for maintaining optimal performance and security standards.
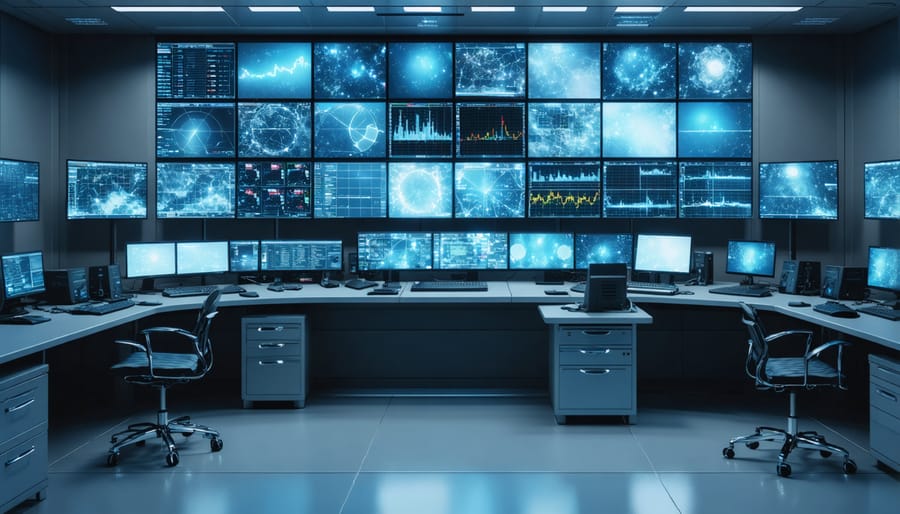
Distributed Energy Resources
Modern infrastructure resilience increasingly relies on decentralized power generation systems to enhance grid stability and maintain critical operations during disruptions. This approach distributes power generation across multiple locations, reducing vulnerability to single-point failures and improving overall system reliability.
Key components of distributed energy resources include solar arrays, wind turbines, micro-turbines, and fuel cells, complemented by advanced energy storage solutions such as lithium-ion batteries and thermal storage systems. These technologies enable facilities to operate independently during grid outages while contributing to regular power supply during normal operations.
Recent implementations demonstrate significant benefits. For example, a medical center in California reduced its vulnerability to wildfire-related power outages by installing a microgrid system, combining solar generation with battery storage. This installation maintained critical operations during three major outages in 2022, potentially saving lives and millions in operational losses.
The integration of smart controls and automation further enhances these systems’ effectiveness. Advanced monitoring technologies enable real-time load management and predictive maintenance, while artificial intelligence optimizes power distribution based on usage patterns and weather forecasts.
Industry experts recommend a phased approach to implementation, starting with critical infrastructure assessment and gradually expanding capacity. This strategy allows organizations to optimize their investments while building system resilience over time. Successful deployment requires careful consideration of local regulations, grid interconnection requirements, and maintenance protocols to ensure long-term sustainability and reliability.
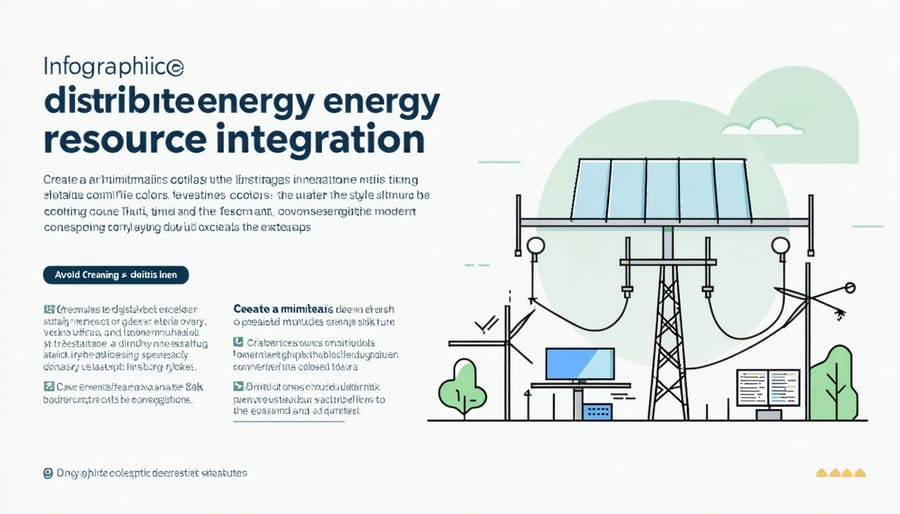
Self-Healing Networks
Self-healing networks represent a groundbreaking advancement in infrastructure resilience, incorporating sophisticated fault detection systems and automated recovery protocols. These intelligent systems continuously monitor network performance, identify potential failures, and initiate corrective actions without human intervention, significantly reducing downtime and maintenance costs.
At the heart of self-healing infrastructure are advanced sensors and artificial intelligence algorithms that work in tandem to create a responsive and adaptive network. These systems excel in power grid management, where they can instantly reroute power flows around damaged sections, preventing cascading failures and maintaining service continuity.
Recent implementations have demonstrated remarkable success rates, with some networks achieving up to 95% autonomous fault resolution. For example, the Metropolitan Power Authority’s smart grid implementation reduced system recovery time from hours to mere minutes, resulting in significant improvements in service reliability and customer satisfaction.
Key components of self-healing networks include:
– Real-time monitoring systems utilizing IoT sensors
– Advanced analytics platforms for predictive maintenance
– Automated switching and rerouting capabilities
– Machine learning algorithms for pattern recognition
– Distributed control systems for localized decision-making
The integration of these technologies enables infrastructure to respond dynamically to various challenges, from equipment failures to cyber threats. Modern self-healing systems can identify potential issues before they become critical, initiating preventive measures that extend asset lifespans and optimize operational efficiency.
Industry experts emphasize that successful implementation requires careful planning and coordination between multiple stakeholders. Organizations must consider factors such as system compatibility, data security, and regulatory compliance when deploying self-healing capabilities. While the initial investment may be substantial, the long-term benefits in terms of reduced maintenance costs and improved service reliability make it an increasingly attractive solution for infrastructure resilience.
Implementation Framework
Risk Assessment Protocols
Effective risk assessment protocols form the cornerstone of infrastructure resilience planning, combining systematic evaluation methods with advanced analytical tools to identify potential vulnerabilities. The process typically begins with a comprehensive asset inventory, followed by threat identification, vulnerability analysis, and consequence assessment.
The primary assessment framework involves three key phases: identification, analysis, and evaluation. During the identification phase, teams document critical infrastructure components and their interdependencies. This includes physical assets, operational systems, and digital networks that could impact overall functionality.
Vulnerability analysis employs both qualitative and quantitative methods to assess potential weaknesses. Modern assessment tools utilize sophisticated modeling software to simulate various threat scenarios, including natural disasters, cyber attacks, and structural failures. These simulations help quantify potential impacts and establish priority areas for reinforcement.
Risk matrices are commonly deployed to categorize threats based on likelihood and potential impact. This approach enables organizations to allocate resources effectively, focusing on high-probability, high-impact risks first. Regularly scheduled assessments, typically conducted annually or after significant system modifications, ensure continued relevance of protective measures.
Industry best practices emphasize the importance of cross-functional team involvement in risk assessment. Engineers, operations personnel, security specialists, and management representatives should collaborate to provide comprehensive perspectives on potential vulnerabilities.
Documentation and standardization of assessment protocols ensure consistency across different infrastructure components and assessment periods. This includes maintaining detailed records of identified risks, implemented mitigation measures, and their effectiveness over time. Regular updates to assessment protocols reflect emerging threats and technological advancements, maintaining the relevance and effectiveness of resilience strategies.
Cost-Benefit Analysis
Investing in infrastructure resilience requires careful evaluation of both immediate costs and long-term benefits. Studies indicate that every dollar invested in resilience measures can yield between $4 and $11 in future savings through reduced damage repair costs and minimized operational disruptions.
The initial capital expenditure for implementing resilience measures typically includes structural reinforcements, redundant systems, and advanced monitoring technologies. While these costs can be substantial, ranging from 2% to 15% of total project value, they must be weighed against potential losses from infrastructure failure or service interruption.
Research from the World Bank demonstrates that infrastructure failures cost businesses billions annually in lost productivity. For example, power outages in major urban centers can cost up to $1 million per hour in economic losses. Resilient infrastructure significantly reduces these risks while extending asset life cycles and decreasing maintenance costs.
Return on investment calculations should consider:
– Reduced insurance premiums
– Lower maintenance and repair costs
– Minimized downtime during extreme events
– Extended infrastructure lifespan
– Enhanced operational efficiency
– Improved stakeholder confidence
Case studies from recent natural disasters reveal that organizations with resilient infrastructure recovered 60% faster and spent 40% less on repairs compared to those without adequate resilience measures. This data strongly supports the economic viability of infrastructure resilience investments, particularly when evaluated over the complete lifecycle of assets.
Regulatory Compliance
Infrastructure resilience standards are governed by an evolving framework of regulations that continues to adapt to emerging challenges and technological advancement. The International Building Code (IBC) and ASCE 7 standards form the foundation of current compliance requirements, establishing minimum criteria for structural integrity and performance under various stress conditions.
Recent updates to FEMA guidelines have introduced more stringent requirements for critical infrastructure, particularly in regions prone to natural disasters. These specifications now mandate enhanced structural redundancy and the integration of smart monitoring systems, reflecting the industry’s shift toward proactive risk management.
As the energy transition accelerates, regulatory bodies are implementing new resilience requirements specifically addressing grid infrastructure and renewable energy systems. The North American Electric Reliability Corporation (NERC) has established Critical Infrastructure Protection (CIP) standards, which now include cybersecurity protocols and physical security measures for energy facilities.
Looking ahead, upcoming regulations will likely focus on climate adaptation strategies, requiring infrastructure projects to demonstrate long-term resilience against projected environmental changes. The EU’s taxonomy for sustainable activities and similar frameworks emerging globally are setting new benchmarks for resilience in infrastructure development.
Organizations must maintain comprehensive documentation of their compliance efforts, including regular assessments, maintenance records, and emergency response plans. This documentation serves both as evidence of regulatory adherence and as a foundation for continuous improvement in resilience strategies.
As we look toward the future of energy infrastructure resilience, the key principles and strategies discussed throughout this article become increasingly critical. The convergence of climate change impacts, cybersecurity threats, and aging infrastructure presents both challenges and opportunities for the construction industry. Successfully implementing resilient infrastructure requires a multi-faceted approach that combines robust physical structures, advanced technology integration, and adaptable management systems.
The evidence clearly shows that organizations investing in infrastructure resilience achieve significant long-term benefits, including reduced maintenance costs, improved operational efficiency, and enhanced system reliability. Case studies from recent natural disasters and cyber incidents demonstrate that resilient infrastructure can mean the difference between quick recovery and prolonged disruption to essential services.
Looking ahead, several trends will shape the future of infrastructure resilience. Artificial intelligence and machine learning are revolutionizing predictive maintenance and real-time monitoring capabilities. The integration of distributed energy resources and microgrids is creating more flexible and redundant power systems. Additionally, advances in materials science are producing more durable and adaptive construction materials that can better withstand environmental stresses.
However, challenges remain. The investment required for infrastructure upgrades can be substantial, and organizations must carefully balance immediate costs against long-term benefits. Regulatory frameworks continue to evolve, requiring ongoing attention to compliance and adaptation to new standards. The increasing complexity of integrated systems also demands greater expertise in both construction and technology management.
Success in building resilient infrastructure will depend on continued collaboration between industry stakeholders, government agencies, and technology providers. Organizations must maintain a proactive stance, regularly assessing vulnerabilities and updating resilience strategies to address emerging threats. Training and workforce development will play crucial roles in ensuring the industry has the expertise needed to implement and maintain resilient systems.
The path forward requires commitment to innovation, investment in proven solutions, and dedication to continuous improvement. By embracing these principles, the construction industry can create infrastructure that not only survives challenges but thrives in the face of adversity.