Zero-energy buildings represent the pinnacle of sustainable architecture, combining advanced renewable energy systems with exceptional energy efficiency to produce as much energy as they consume annually. As construction costs decline and global environmental regulations tighten, these high-performance structures have evolved from ambitious experiments to commercially viable solutions deployed worldwide.
The integration of smart microgrids with zero-energy buildings creates a powerful synergy that revolutionizes how we generate, distribute, and consume energy. By incorporating on-site renewable generation, energy storage systems, and intelligent load management, these buildings not only achieve net-zero energy status but also strengthen grid resilience and reduce peak demand pressures on conventional power infrastructure.
Leading construction firms and developers are increasingly recognizing that zero-energy buildings deliver compelling returns on investment through dramatically reduced operating costs, enhanced property values, and improved occupant satisfaction. As we navigate the critical challenges of climate change and energy security, zero-energy buildings equipped with microgrid capabilities represent a proven, scalable solution that transforms our built environment while creating healthier, more sustainable communities.
Understanding Zero Energy Building Design
Passive Design Strategies
Passive design strategies form the foundation of zero energy buildings, significantly reducing energy demand before active systems are considered. Building orientation plays a crucial role, with optimal positioning allowing structures to maximize solar heat gain in winter and minimize it in summer. South-facing windows (in the Northern hemisphere) can capture up to 40% more solar energy than other orientations, reducing heating requirements during colder months.
High-performance insulation is essential for maintaining stable indoor temperatures. Modern buildings utilize advanced materials such as vacuum-insulated panels and aerogels, achieving R-values three to four times higher than traditional insulation methods. The building envelope must be thoroughly sealed to prevent thermal bridges and air leakage, typically resulting in a 60-70% reduction in heating and cooling loads.
Natural ventilation strategies, including stack effect and cross-ventilation, can eliminate the need for mechanical cooling in moderate climates. Strategic placement of operable windows, coupled with automated control systems, optimizes airflow patterns while maintaining indoor comfort levels. Leading designs incorporate wind towers and solar chimneys to enhance passive ventilation effectiveness.
Daylighting techniques reduce artificial lighting requirements while improving occupant well-being. Light shelves, clerestory windows, and solar tubes distribute natural light deep into building interiors, potentially reducing lighting energy consumption by 50-80%. Advanced glazing systems with dynamic tinting capabilities help balance daylighting benefits with solar heat gain control, ensuring year-round performance optimization.
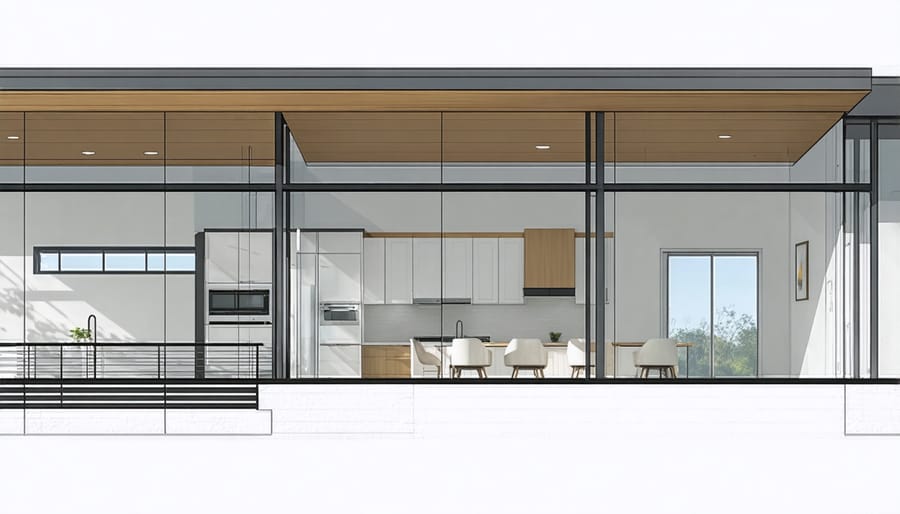
Active Energy Systems
Active energy systems form the backbone of zero energy buildings, integrating renewable energy technologies with smart building management systems to achieve optimal performance. Solar photovoltaic arrays remain the primary renewable energy source, typically complemented by technologies such as ground-source heat pumps and wind turbines where site conditions permit.
Modern zero energy buildings employ sophisticated energy storage solutions, including advanced battery systems and thermal storage, to address intermittency issues inherent in renewable sources. These storage systems enable buildings to maintain consistent power supply during peak demand periods and adverse weather conditions.
Building-integrated photovoltaics (BIPV) represent an increasingly popular solution, incorporating solar generation capabilities directly into building materials such as roof tiles, facades, and windows. This approach maximizes available surface area while maintaining architectural aesthetics.
Energy management platforms utilizing artificial intelligence and machine learning algorithms continuously optimize system performance by analyzing real-time data from thousands of sensors. These systems automatically adjust HVAC operations, lighting levels, and power distribution based on occupancy patterns, weather conditions, and energy pricing signals.
The integration of microgrids enables buildings to operate independently when necessary while maintaining grid connection for reliability and potential energy trading opportunities. This hybrid approach ensures resilience during grid outages while supporting broader community energy objectives.
Microgrid Integration in Zero Energy Buildings
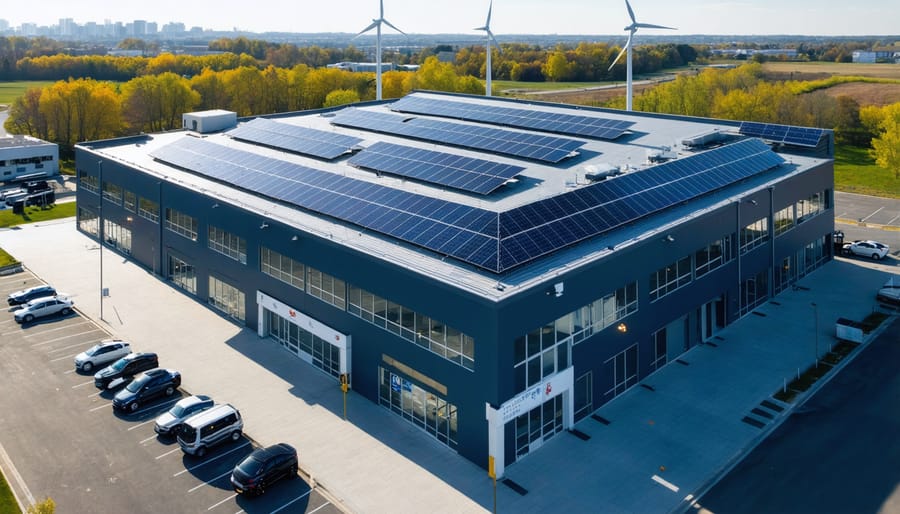
On-site Energy Generation
On-site energy generation forms the cornerstone of zero energy building design, with solar photovoltaic (PV) systems leading the way as the most widely adopted technology. Modern solar installations typically achieve conversion efficiencies between 18-22%, with bifacial panels offering additional energy capture through reflected light on their rear surfaces.
Rooftop solar arrays remain the primary choice for most projects, but building-integrated photovoltaics (BIPV) are gaining traction by incorporating solar cells into building materials like windows, facades, and roof tiles. This dual-purpose approach maximizes available surface area while maintaining architectural aesthetics.
Wind energy systems, while less common in urban settings, prove valuable for rural or coastal projects. Small-scale wind turbines, particularly vertical axis models, can complement solar installations by generating power during nighttime hours and overcast conditions. However, careful site analysis is essential to ensure adequate wind resources and address potential noise concerns.
Other renewable technologies finding application include ground-source heat pumps for thermal energy and micro-hydroelectric systems where water resources permit. Combined heat and power (CHP) systems using renewable biofuels can provide both electrical power and thermal energy, particularly beneficial in colder climates.
Energy storage systems, typically lithium-ion batteries, play a crucial role in managing intermittent renewable generation. Advanced energy management systems optimize the interaction between generation sources, storage, and building loads, ensuring reliable power supply while maximizing self-consumption of generated energy.
Success in on-site generation requires careful consideration of local climate conditions, available space, building orientation, and regulatory requirements. Regular maintenance and monitoring ensure optimal system performance and longevity.
Energy Storage Solutions
Effective energy storage systems are crucial components in achieving true zero energy building status, enabling the consistent management of renewable energy resources throughout varying demand periods. Modern energy storage technologies have evolved significantly, offering building operators multiple options for maintaining energy independence.
Lithium-ion battery systems remain the most widely adopted storage solution, providing high energy density and rapid response capabilities. These systems typically operate at 85-95% efficiency and can be scaled to meet various building sizes. Advanced battery management systems (BMS) optimize charging cycles and extend battery life while ensuring safe operation.
Thermal energy storage presents another viable option, particularly for buildings with significant heating and cooling demands. Phase change materials (PCMs) and ice storage systems can shift cooling loads to off-peak hours, reducing strain on the electrical system during peak demand periods.
Flow batteries are gaining traction in commercial applications, offering advantages like independent scaling of power and energy capacity, longer cycle life, and reduced fire risk compared to traditional lithium-ion systems. While initial costs remain higher, their operational flexibility makes them increasingly attractive for large-scale implementations.
Emerging technologies include hydrogen storage systems and gravity-based solutions, though these are currently less common in building applications. Integration of multiple storage types, known as hybrid storage systems, often provides the most robust solution, allowing buildings to optimize energy management based on varying seasonal and daily demands while maintaining system reliability and efficiency.
Real-World Implementation Success Stories
Commercial Building Case Study
The Bullitt Center in Seattle, Washington, stands as a landmark achievement in commercial zero energy building design and operation. Completed in 2013, this six-story, 52,000-square-foot commercial office building is widely recognized as one of the world’s most energy-efficient commercial structures.
The building achieves its zero energy status through a combination of advanced design strategies and innovative technologies. Its rooftop solar array, consisting of 575 photovoltaic panels, generates 60% more electricity than the building consumes annually. The structure’s design maximizes natural daylight through strategic window placement and light shelves, reducing artificial lighting needs by 80% compared to conventional office buildings.
Energy consumption is minimized through a ground-source heat pump system for heating and cooling, triple-paned windows, and an ultra-tight building envelope with R-40 walls and R-60 roof insulation. The building’s energy use intensity (EUI) averages 12 kBtu/sq ft/year, compared to 60-70 kBtu/sq ft/year for typical office buildings in Seattle.
Performance data from five years of operation demonstrates consistent net-positive energy production, with the building generating approximately 60% more energy than it uses. This surplus energy is fed back into the city’s power grid, effectively making the Bullitt Center a clean energy provider for its neighborhood. The project’s success has influenced building codes and demonstrated the commercial viability of zero energy buildings in urban environments.
Institutional Building Case Study
The Bullitt Center in Seattle, Washington, stands as a pioneering example of zero energy achievement in institutional buildings. Completed in 2013, this six-story, 52,000-square-foot commercial building is recognized as one of the world’s most energy-efficient structures, consistently producing more energy than it consumes.
The building’s success stems from its integrated design approach, combining passive and active strategies. A photovoltaic array consisting of 575 panels generates 60% more electricity than required for operations, despite Seattle’s notably cloudy climate. The structure’s orientation maximizes natural daylight, while automated external blinds and light sensors optimize solar gain and reduce cooling loads.
Energy consumption is minimized through several innovative features, including ground-source heat pumps, a high-performance building envelope with triple-paned windows, and advanced energy monitoring systems. The building achieves an impressive Energy Use Intensity (EUI) of 12 kBtu/sq ft/year, compared to the national average of 93 kBtu/sq ft/year for office buildings.
Post-occupancy data reveals that behavioral factors significantly influence performance. The building’s success relies heavily on occupant engagement through real-time energy dashboards and regular training sessions. Operating costs have decreased by 60% compared to conventional buildings, demonstrating the financial viability of zero energy institutional projects.
The Bullitt Center serves as a replicable model, proving that zero energy goals are achievable even in challenging climate conditions with proper planning and implementation.
Economic and Environmental Benefits
Zero energy buildings (ZEBs) demonstrate compelling economic advantages through significant long-term cost reductions and enhanced environmental performance. While initial construction costs typically run 5-15% higher than conventional buildings, the return on investment (ROI) is increasingly attractive, with most projects achieving payback periods of 7-12 years through reduced operational expenses.
Studies from the U.S. Department of Energy show that ZEBs can reduce energy costs by 60-90% compared to standard buildings, translating to annual savings of $1.50-$2.50 per square foot in commercial applications. These savings are particularly impactful when combined with energy efficient retrofits in existing structures.
The environmental benefits are equally substantial. A typical 50,000-square-foot zero energy commercial building prevents approximately 500-700 metric tons of CO2 emissions annually – equivalent to removing 100 cars from the road. Beyond carbon reduction, ZEBs contribute to improved air quality, reduced water consumption, and decreased strain on local power infrastructure.
Market analysis indicates that ZEBs command premium resale values, typically 7-12% higher than comparable conventional buildings. Additionally, these buildings often qualify for tax incentives, grants, and other financial benefits that can offset initial construction costs. Operating costs remain predictable and stable, protecting owners from energy price volatility.
The integration of renewable energy systems, particularly through microgrids, enhances resilience while providing additional revenue streams through grid services and excess energy sales. Furthermore, ZEBs consistently demonstrate higher occupancy rates and increased worker productivity, with studies showing up to 18% improvement in employee performance in zero energy workspaces.
As technology costs continue to decrease and energy prices rise, the financial case for zero energy buildings strengthens annually, making them an increasingly attractive option for forward-thinking developers and building owners.
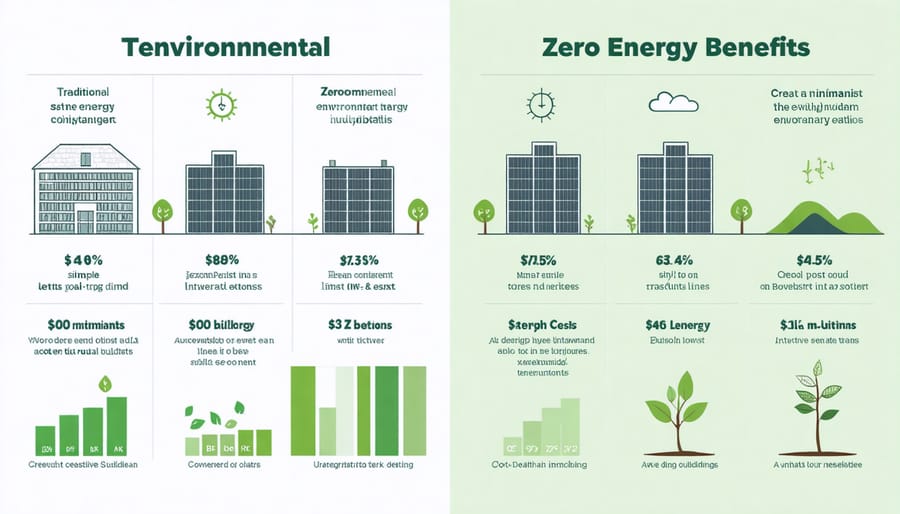
Zero energy buildings represent a crucial evolution in sustainable construction, marking a significant shift toward carbon-neutral architecture and development. As demonstrated throughout this analysis, the integration of advanced building technologies, renewable energy systems, and intelligent energy management solutions has made net-zero energy consumption both technically feasible and economically viable.
The success of implemented projects worldwide proves that zero energy buildings are not merely aspirational concepts but practical solutions for modern construction. From commercial complexes to residential developments, these buildings consistently demonstrate substantial operational cost savings while maintaining optimal occupant comfort and functionality.
Looking ahead, the zero energy building sector is poised for exponential growth, driven by strengthening regulatory frameworks, advancing technology, and increasing market demand. The continuous improvement in renewable energy efficiency, coupled with declining implementation costs, will likely accelerate adoption rates across various building sectors.
However, achieving widespread implementation requires ongoing collaboration between architects, engineers, contractors, and policymakers. Industry professionals must continue to prioritize integrated design approaches, emphasizing early-stage planning and comprehensive energy modeling. Additionally, the expansion of smart grid technologies and energy storage solutions will play a pivotal role in optimizing building performance and grid integration.
As climate change concerns intensify and energy costs rise, zero energy buildings will increasingly become the standard rather than the exception in construction. This transition represents not just an environmental imperative but a fundamental transformation in how we conceive, design, and operate our built environment.