Wind energy construction represents one of the fastest-growing sectors in renewable energy construction, with global capacity expanding by 93 GW in 2023 alone. This surge demands precise engineering, substantial capital investment, and sophisticated project management expertise to execute successfully.
Modern wind farm construction encompasses far more than turbine installation – it requires comprehensive site analysis, advanced foundation engineering, and intricate logistics coordination across expansive geographical areas. Project managers must navigate complex regulatory frameworks while orchestrating the seamless integration of massive components, often in challenging terrains and weather conditions.
The economic implications are equally significant, with utility-scale wind projects typically representing investments of $200-400 million per 100MW of capacity. This capital-intensive nature, combined with strict technical requirements and environmental considerations, makes wind energy construction one of the most demanding segments in contemporary infrastructure development.
For construction professionals and project managers, mastering wind energy construction requires deep understanding of aerodynamic principles, structural engineering, and emerging technologies in turbine design. Success depends on balancing these technical demands with environmental impact assessments, community engagement, and precise project scheduling to ensure optimal return on investment.
Site Assessment and Planning Optimization
Wind Resource Analysis and Site Selection
Wind resource analysis forms the foundation of successful wind energy projects, requiring comprehensive assessment of multiple environmental and geographical factors. The process begins with detailed wind measurements conducted over 12-24 months using meteorological towers equipped with anemometers at various heights. These measurements capture critical data including wind speed, direction, frequency, and turbulence intensity.
Site selection involves evaluating both wind characteristics and practical considerations. Optimal locations typically feature average wind speeds exceeding 6.5 meters per second at hub height, with consistent wind patterns throughout the year. Topographical features, such as ridgelines and open plains, can enhance wind resources by creating natural wind tunnels or exposure to prevailing winds.
Modern wind resource assessment employs sophisticated tools including LiDAR technology, computational fluid dynamics (CFD) modeling, and historical weather data analysis. These methods help create detailed wind resource maps and energy yield predictions, essential for project feasibility studies and financial planning.
Key factors in site evaluation include:
– Grid connection accessibility
– Environmental impact considerations
– Land availability and terrain suitability
– Transportation access for construction equipment
– Local zoning regulations and permits
– Community acceptance and stakeholder concerns
Advanced micrositing techniques determine precise turbine positions to maximize energy capture while minimizing wake effects between turbines. This optimization process considers factors such as terrain roughness, obstacle effects, and array efficiency to ensure optimal project performance throughout its operational lifetime.
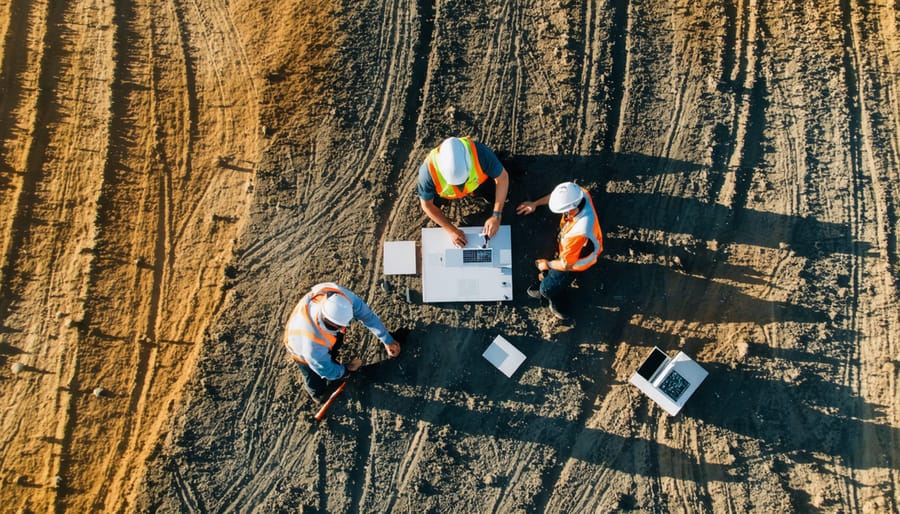
Environmental Impact Studies and Compliance
Environmental impact studies are crucial prerequisites for wind energy construction projects, typically beginning 12-24 months before construction. These assessments evaluate potential effects on wildlife, particularly bird and bat populations, noise levels, visual impact, and local ecosystems.
Key environmental compliance requirements include conducting thorough wildlife surveys, analyzing migration patterns, and assessing potential interference with local habitats. Project developers must work closely with environmental consultants to perform site-specific studies that meet both federal and state regulations.
The assessment process typically involves:
– Baseline environmental surveys
– Bird and bat mortality risk assessments
– Shadow flicker analysis
– Noise impact studies
– Visual impact assessments
– Cultural resource surveys
– Wetland delineation
Compliance documentation must demonstrate adherence to various regulatory frameworks, including the National Environmental Policy Act (NEPA), Endangered Species Act, and state-specific environmental regulations. Many projects require ongoing monitoring during operation, particularly for wildlife impacts.
Successful environmental compliance often involves implementing mitigation measures such as:
– Strategic turbine placement to minimize wildlife impact
– Installation of wildlife detection and deterrent systems
– Establishment of buffer zones around sensitive areas
– Development of habitat conservation plans
– Implementation of construction timing restrictions during breeding seasons
Project teams must maintain detailed documentation throughout the environmental review process and be prepared for regulatory agency inspections during both construction and operational phases.
Infrastructure Development and Construction Phases
Foundation and Access Road Construction
The foundation and access road infrastructure represents a critical phase in wind energy construction, requiring precise engineering and robust construction methodologies. These foundational elements must support turbine structures weighing hundreds of tons while facilitating efficient renewable energy integration into existing power grids.
Foundation construction typically begins with extensive soil testing and geotechnical analysis to determine optimal foundation design. The most common foundation type is the spread footer design, requiring excavation depths of 2-3 meters and reinforced concrete volumes ranging from 300-800 cubic meters per turbine. Steel reinforcement cages, precisely engineered to handle both compression and tension forces, are assembled on-site and positioned before concrete pouring commences.
Access road construction demands equally rigorous specifications to support heavy equipment transport. These roads typically require a minimum width of 4.5 meters, with additional clearance on curves, and must be capable of supporting vehicles weighing up to 150 tons. The road base usually consists of compacted aggregate layers, with thickness varying between 300-500mm depending on soil conditions and expected loads.
Key technical specifications include:
– Concrete strength requirements of 30-40 MPa
– Foundation settling tolerance of less than 3mm per meter
– Access road grade limitations of 8-10%
– Turning radius requirements of 50-60 meters for transport vehicles
– Soil bearing capacity minimum of 25,000 kg/m²
Construction sequencing typically follows a strict timeline, with foundation curing periods of 28 days before turbine installation can commence. Weather conditions, particularly temperature and precipitation, must be carefully monitored during concrete pouring operations to ensure proper curing and structural integrity.
Turbine Assembly and Installation Logistics
The assembly and installation of wind turbines represents one of the most complex phases in wind energy construction, requiring precise coordination of specialized equipment, skilled personnel, and favorable weather conditions. The process typically begins with the delivery of major components, including tower sections, nacelles, and rotor blades, which must be carefully sequenced to minimize on-site storage requirements and optimize installation efficiency.
Heavy-duty cranes, typically with capacities exceeding 600 tons, are essential for the vertical assembly process. The tower sections are installed first, followed by the nacelle, which houses the generator and gearbox. The most challenging aspect often involves the attachment of rotor blades, as this operation requires minimal wind conditions and expert precision to manage the aerodynamic loads during installation.
Weather windows play a crucial role in installation scheduling, with wind speeds typically needing to remain below 12 meters per second for safe crane operations. This requirement often necessitates careful planning around seasonal weather patterns and can significantly impact project timelines. Installation teams must maintain 24-hour weather monitoring capabilities and be prepared to pause operations when conditions become unfavorable.
Transportation logistics present another significant challenge, particularly for modern turbines with blade lengths exceeding 65 meters. Special routing considerations, including road modifications and temporary infrastructure improvements, are often necessary to accommodate these oversized components. Some projects require specialized transport vehicles with active steering systems to navigate tight turns and challenging terrain.
Quality control during assembly is paramount, with particular attention paid to bolt tensioning, electrical connections, and mechanical alignments. Each connection point must meet strict torque specifications, and comprehensive testing protocols are implemented before commissioning. Modern turbines often incorporate sophisticated monitoring systems that must be properly calibrated during installation to ensure optimal performance and reliability throughout the turbine’s operational life.
These complex operations require experienced project managers who can coordinate multiple contractors, maintain strict safety protocols, and adapt to changing site conditions while keeping the project on schedule and within budget.
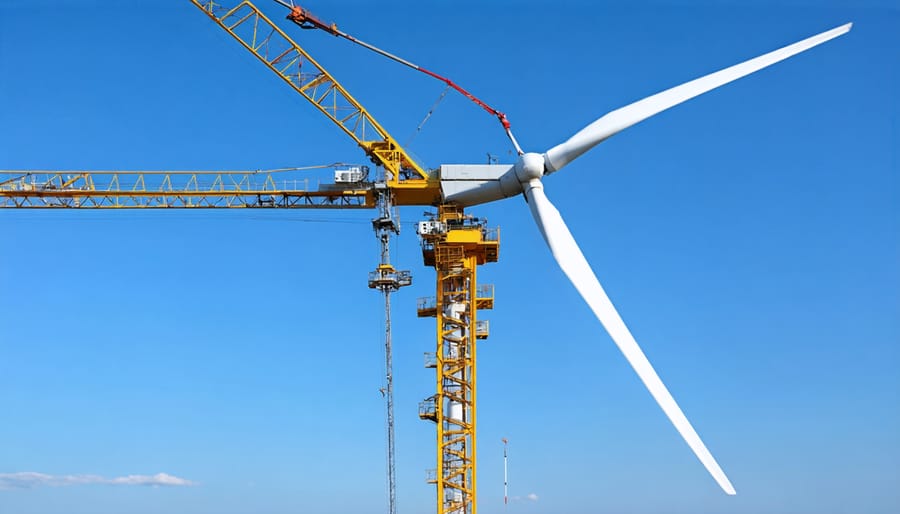
Grid Integration and Power Systems
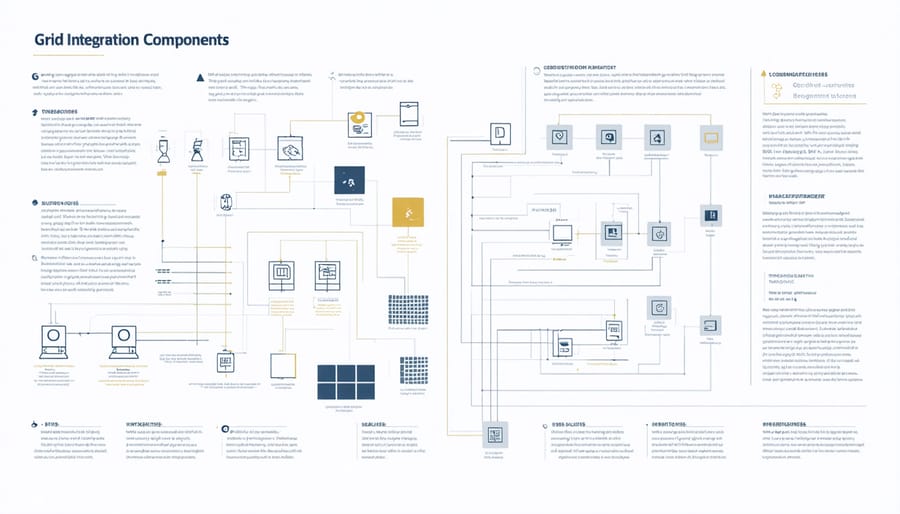
Electrical Infrastructure Requirements
The electrical infrastructure requirements for wind energy projects demand careful consideration of power transmission and distribution components. Central to this infrastructure are the grid integration systems that facilitate seamless power transfer from turbines to end-users.
Primary components include step-up transformers at each turbine base, typically raising voltage from 690V to 33kV, and main substation transformers that further increase voltage levels for long-distance transmission. Underground collection systems connect individual turbines to the main substation through medium-voltage cables, requiring careful routing and installation to minimize environmental impact.
Protection systems are essential, incorporating circuit breakers, disconnectors, and sophisticated monitoring equipment to safeguard against electrical faults and overloads. Modern wind farms also require fiber optic networks for real-time monitoring and control, integrating SCADA systems for optimal performance management.
Compliance with grid codes is paramount, necessitating power quality equipment such as reactive power compensation devices and harmonic filters. The infrastructure must maintain stable frequency and voltage levels while managing intermittent power generation characteristics inherent to wind energy systems.
Construction specifications must account for future capacity expansion, incorporating appropriate sizing of switchgear, transformers, and transmission lines to accommodate potential additional turbines or storage systems.
Smart Grid Integration Strategies
Modern wind energy facilities require sophisticated integration strategies to effectively connect with existing power infrastructure. The implementation of smart grid technology has revolutionized how wind farms interact with power distribution networks, enabling real-time monitoring and dynamic load balancing.
Key integration components include advanced SCADA systems, power conditioning equipment, and intelligent switching mechanisms. These elements work in concert to manage voltage fluctuations, maintain grid stability, and optimize power delivery during varying wind conditions. Modern wind facilities employ sophisticated power electronics and control systems to ensure seamless integration with existing infrastructure.
Grid connection strategies typically incorporate redundant transmission pathways and advanced forecasting systems to predict and manage power output effectively. Protective relay systems and circuit breakers are strategically positioned to safeguard both the wind facility and the broader grid infrastructure from potential disruptions or failures.
Construction professionals must coordinate closely with utility providers during the integration phase, ensuring compliance with grid codes and interconnection requirements. This includes implementing appropriate transformer configurations, installing power quality monitoring equipment, and establishing reliable communication protocols between the wind facility and grid operators.
Recent innovations in battery storage systems have further enhanced grid integration capabilities, allowing wind facilities to store excess energy during peak production periods and release it during low-wind conditions, thereby maintaining consistent power supply to the grid.
Project Management and Cost Control
Timeline Management and Risk Mitigation
Effective timeline management in wind energy construction requires a robust framework of scheduling tools and risk mitigation strategies. Project managers typically employ critical path method (CPM) scheduling, identifying key milestones such as foundation construction, turbine delivery, and grid connection deadlines. Weather windows must be carefully planned, particularly for offshore installations where sea conditions can significantly impact crane operations and vessel availability.
Risk management begins with comprehensive site assessments and contingency planning. Construction teams should maintain flexible schedules that account for seasonal weather patterns and potential supply chain disruptions. Buffer periods between major installation phases help absorb unexpected delays without compromising overall project timelines.
Regular monitoring of key performance indicators (KPIs) and early warning systems helps identify potential schedule slippages before they become critical. This includes tracking equipment delivery schedules, monitoring weather forecasts, and maintaining close communication with suppliers and subcontractors.
Common risk mitigation strategies include:
– Establishing multiple supplier relationships to reduce dependency
– Implementing weather monitoring systems with clear go/no-go criteria
– Developing detailed logistics plans with alternative transportation routes
– Maintaining emergency response protocols for equipment failures
– Creating modular construction approaches that allow for parallel work streams
Success in timeline management often depends on the integration of digital project management tools and real-time communication systems. These enable quick decision-making and help teams adapt to changing conditions while maintaining project momentum.
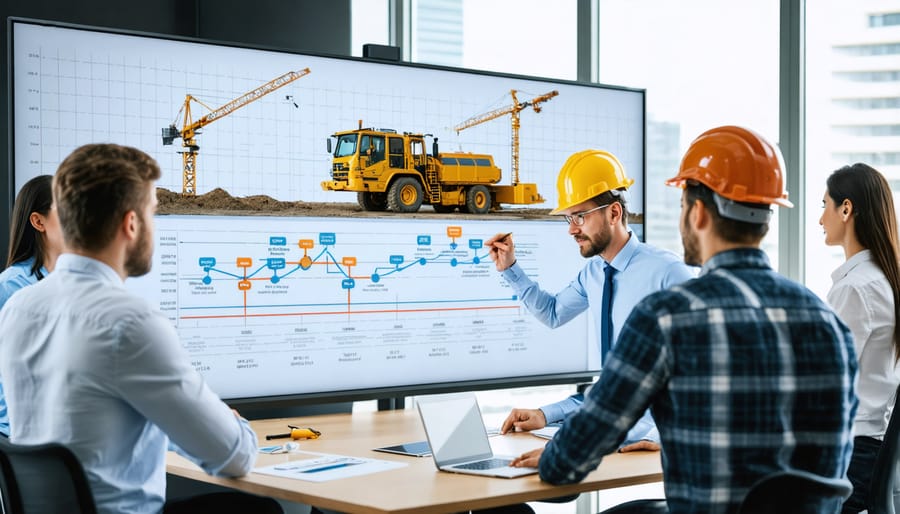
Cost Optimization Techniques
Implementing effective cost optimization strategies is crucial for successful wind energy construction projects. By integrating sustainable construction practices with smart financial planning, project managers can significantly reduce expenses while maintaining quality standards.
Strategic procurement plays a vital role in cost control. Bulk purchasing of materials, particularly for multiple turbine installations, can lead to substantial savings. Establishing long-term relationships with reliable suppliers often results in preferential pricing and improved payment terms.
Modular construction techniques have proven highly effective in reducing both labor costs and construction timelines. Pre-fabricated components can be assembled off-site under controlled conditions, minimizing weather-related delays and reducing on-site labor requirements.
Optimizing construction schedules through careful planning of crane operations is essential, as crane rental represents a significant project cost. Coordinating multiple turbine installations within the same rental period maximizes equipment utilization and reduces standby charges.
Advanced modeling software for site optimization helps determine the most cost-effective turbine placement, considering factors like wind resource quality, foundation requirements, and access road construction costs. This data-driven approach prevents expensive modifications during construction and ensures optimal energy production capacity.
Regular maintenance planning during the construction phase can prevent costly repairs and downtime later. Installing monitoring systems and implementing preventive maintenance protocols from the outset provides long-term cost benefits throughout the project lifecycle.
Case Study: Successful Wind Farm Implementation
The Glenrock Wind Farm project in Wyoming stands as a prime example of successful wind energy construction implementation. Completed in 2019, this 150MW facility demonstrates how careful planning and execution can overcome complex construction challenges while meeting environmental and community requirements.
The project team faced several significant challenges, including difficult terrain, extreme weather conditions, and the need to minimize environmental impact in sensitive areas. The construction phase spanned 18 months and involved the installation of 66 wind turbines across 30,000 acres of mixed-use land.
Key success factors included comprehensive geotechnical surveys that informed foundation design and construction methods. The project team utilized innovative approaches to overcome soil stability issues, implementing specialized foundation systems that reduced concrete usage by 20% while maintaining structural integrity.
Transportation logistics proved crucial to the project’s success. The team developed a custom route planning system to facilitate the movement of oversized components, including 62-meter blades and tower sections weighing up to 70 tons. This system reduced transportation-related delays by 35% compared to industry averages.
The construction team employed advanced project management techniques, including real-time progress tracking and dynamic scheduling. This approach allowed for rapid adaptation to changing weather conditions and equipment availability, resulting in completion three weeks ahead of schedule and 5% under budget.
Community engagement played a vital role in the project’s success. The construction team implemented a local hiring program that provided employment for 120 regional workers and established partnerships with local contractors. Regular community updates and site visits helped maintain positive relationships with stakeholders throughout the construction phase.
Post-completion analysis revealed several noteworthy achievements: zero lost-time accidents during construction, 98% uptime during the first year of operation, and successful integration with the regional power grid. The project now serves as a model for future wind farm developments, demonstrating how effective planning, innovative construction techniques, and stakeholder engagement can deliver successful renewable energy infrastructure.
Wind energy construction has emerged as a crucial sector in the sustainable development landscape, with significant growth projected in the coming decades. The successful implementation of wind energy projects demands a careful balance of technical expertise, regulatory compliance, and strategic project management. As we’ve explored, the key factors driving successful wind farm construction include comprehensive site assessment, efficient supply chain management, and adherence to stringent safety protocols.
Looking ahead, the wind energy construction sector is poised for remarkable expansion, driven by technological advancements and increasing renewable energy demands. Innovations in turbine design, construction methodologies, and project management tools continue to enhance efficiency and reduce costs. Industry professionals can expect to see greater integration of digital technologies, improved construction techniques, and more sustainable practices in future projects.
For construction professionals and project managers, staying informed about industry best practices, maintaining updated certifications, and fostering relationships with experienced partners will be essential for success in this dynamic field. As wind energy construction continues to evolve, the emphasis on quality, safety, and environmental stewardship will remain paramount, ensuring the delivery of reliable and sustainable energy infrastructure for generations to come.