Revolutionary building materials are reshaping the future of sustainable construction, driven by unprecedented advances in materials science and environmental imperatives. From self-healing concrete incorporating living bacteria to transparent aluminum composites offering structural strength with optical clarity, these innovations promise to transform our built environment. Advanced carbon-fiber reinforced polymers now enable structures that are simultaneously lighter and stronger than traditional steel, while bio-based materials derived from mycelium and agricultural waste are creating truly regenerative building solutions.
The convergence of nanotechnology, biomimicry, and artificial intelligence is accelerating the development of smart materials that adapt to environmental conditions, regulate temperature autonomously, and minimize energy consumption. These next-generation materials not only address current construction challenges but anticipate future demands for resilience, sustainability, and performance. As global construction activity continues to surge, understanding and implementing these revolutionary materials becomes crucial for industry professionals committed to building a more sustainable and efficient future.
Self-Healing Materials Transform Maintenance
Bio-Concrete Technology
Among the most groundbreaking innovative concrete technologies, bio-concrete stands out for its remarkable self-healing capabilities. This revolutionary material incorporates specially selected bacterial spores that remain dormant within the concrete matrix until activated by water infiltration through cracks. When moisture penetrates these fissures, the bacteria spring to life, initiating a biological process that produces limestone (calcium carbonate) to seal the gaps.
The bacterial species, typically Bacillus subtilis or Bacillus cohnii, are encapsulated in protective shells before being mixed into the concrete. These microorganisms can survive for decades within the material, providing long-term healing potential. When activated, they metabolize organic compounds provided in the mixture, generating calcite precipitate that effectively bridges cracks up to 0.8mm wide.
Laboratory testing has demonstrated that bio-concrete can reduce maintenance costs by up to 50% while extending structure lifespan by 30-50%. The material has proven particularly valuable in water-retaining structures, underground construction, and marine applications where crack formation traditionally poses significant challenges. This self-repairing capability not only enhances durability but also contributes to reduced carbon footprint by minimizing the need for repairs and replacement.
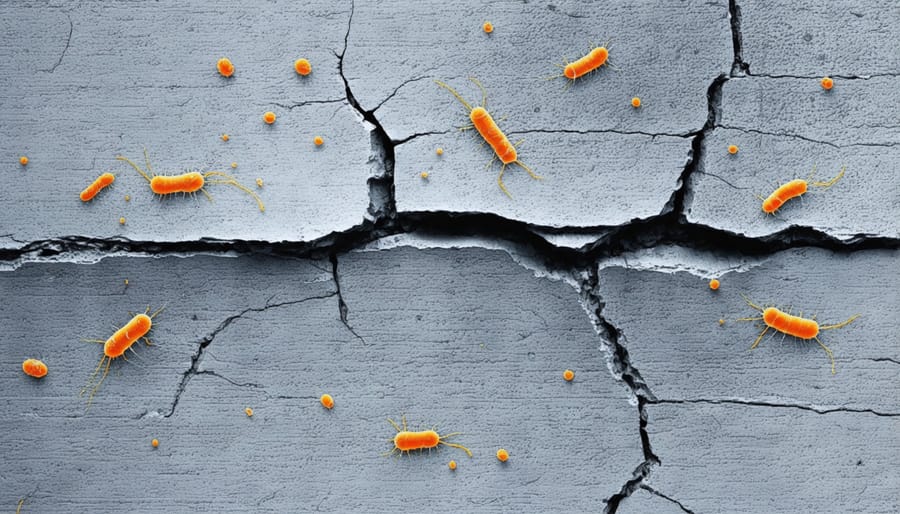
Smart Polymers in Construction
Smart polymers represent a groundbreaking advancement in construction materials, offering unprecedented adaptability and functionality. These innovative materials, particularly shape-memory polymers (SMPs), can change their physical properties in response to environmental stimuli such as temperature, light, or electrical current.
In modern construction applications, SMPs are being integrated into self-repairing building components that can restore their original shape after deformation. For example, when implemented in building facades, these materials can automatically adjust their configuration to optimize energy efficiency and thermal comfort based on external conditions.
The technology has shown remarkable potential in structural applications, where smart polymers can be programmed to respond to stress by increasing their rigidity, thereby enhancing building safety during extreme weather events. Additionally, these materials are being developed for use in smart windows that can automatically adjust their opacity and thermal properties.
Recent developments have also led to the creation of self-healing concrete incorporating smart polymers, which can seal cracks autonomously when they develop. This capability significantly extends the lifespan of concrete structures while reducing maintenance costs and environmental impact.
Construction companies are increasingly adopting these materials for their versatility and potential to create more resilient, adaptive buildings that can respond dynamically to changing environmental conditions.
Carbon-Negative Materials Lead Environmental Innovation
Cross-Laminated Timber Advancements
Cross-laminated timber (CLT) is experiencing a revolutionary transformation in the construction industry, with recent technological advancements significantly enhancing its structural capabilities and applications. Modern manufacturing processes now allow for larger, more precise panels with improved dimensional stability and load-bearing capacity, making CLT suitable for high-rise construction projects previously dominated by steel and concrete.
Notable innovations include the development of hybrid CLT systems that incorporate other materials like steel reinforcements or concrete cores, optimizing structural performance while maintaining sustainability benefits. Advanced adhesive technologies have improved moisture resistance and structural integrity, addressing traditional concerns about wooden construction materials.
Digital fabrication and parametric design tools now enable complex geometries and custom configurations, expanding architectural possibilities. Manufacturers are implementing automated quality control systems using artificial intelligence and machine learning to detect defects and ensure consistent panel quality.
Recent research has also led to enhanced fire-resistance treatments and improved acoustic properties through specialized layering techniques. The integration of smart sensors within CLT panels allows real-time monitoring of structural health, moisture content, and temperature variations, providing valuable data for building maintenance and performance optimization.
These advancements have contributed to CLT’s growing adoption in commercial and residential projects worldwide, with several landmark buildings demonstrating its potential as a primary structural material for sustainable construction.
Biocomposite Materials
Biocomposite materials represent a groundbreaking shift in construction, combining agricultural waste with innovative biological processes to create sustainable building solutions. At the forefront of this revolution is mycelium, the root structure of mushrooms, which can be cultivated with agricultural byproducts to form robust construction materials.
These materials are engineered by combining crop residues such as corn stalks, hemp hurds, or rice hulls with mycelium spores. The fungal network grows through the agricultural waste, creating a natural binding matrix that results in lightweight yet durable building components. The finished products exhibit remarkable properties, including fire resistance, excellent thermal insulation, and acoustic absorption.
Leading construction firms are already implementing biocomposites in non-structural applications such as interior wall panels, insulation, and decorative elements. Recent pilot projects have demonstrated a 40% reduction in carbon footprint compared to traditional materials, while maintaining comparable performance standards.
The manufacturing process is notably energy-efficient, requiring minimal processing and zero chemical additives. The materials are fully biodegradable at end-of-life, addressing the critical issue of construction waste management. Additionally, by utilizing agricultural waste streams, these biocomposites create new value chains for farming communities while reducing the environmental impact of crop residue disposal.
As regulatory frameworks increasingly emphasize sustainable construction practices, biocomposite materials are positioned to become a cornerstone of future building solutions, offering both environmental benefits and practical performance advantages.
Energy-Generating Building Components
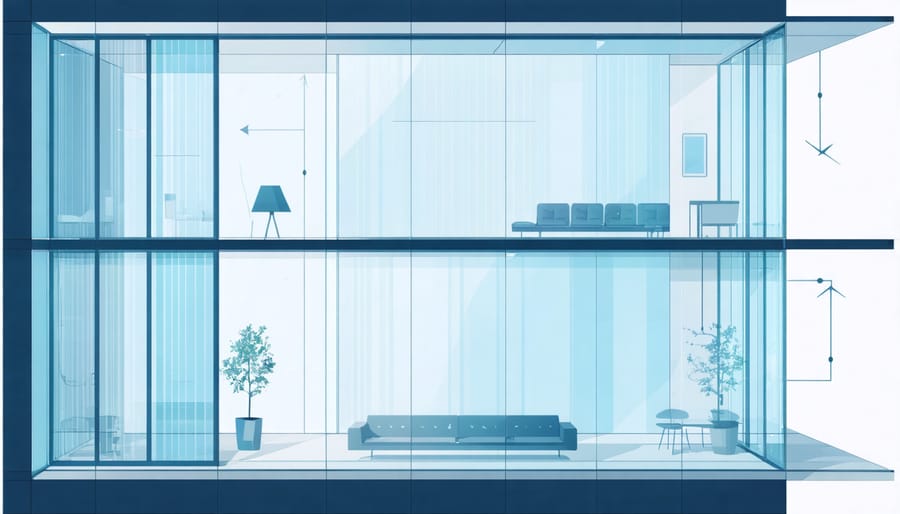
Transparent Solar Cells
Transparent solar cells represent a groundbreaking advancement in building-integrated photovoltaics, transforming standard windows into power-generating surfaces while maintaining their primary function. These innovative materials utilize organic photovoltaic technology to harvest solar energy through selective light absorption, allowing visible light to pass through while capturing ultraviolet and infrared radiation.
Recent developments have achieved transparency levels of up to 70% while maintaining power conversion efficiencies of 5-10%. Michigan State University researchers have demonstrated that when implemented across all glass surfaces of a typical commercial building, these cells could potentially generate up to 40% of the building’s annual energy requirements.
The technology employs specialized molecular engineering, incorporating luminescent solar concentrators (LSCs) that redirect specific wavelengths of light to conventional photovoltaic cells positioned along the window frames. This design ensures optimal power generation without compromising architectural aesthetics or natural lighting.
Leading manufacturers are now producing these cells in various tints and transparency levels, offering architects greater design flexibility. Implementation costs remain higher than traditional windows, but the dual functionality and reduced energy dependency make them increasingly attractive for sustainable building projects, particularly in urban environments where vertical surface area often exceeds roof space.
Piezoelectric Materials
Piezoelectric materials represent a groundbreaking advancement in sustainable building technology, capable of harvesting energy from everyday mechanical stresses and vibrations. These innovative materials generate electrical charges when subjected to mechanical pressure, effectively converting kinetic energy from footsteps, vehicular traffic, and structural movements into usable electricity.
Recent developments have led to the creation of enhanced piezoelectric composites that can be integrated into flooring systems, structural elements, and facade components. For instance, high-traffic areas like building entrances, corridors, and public spaces can be fitted with piezoelectric tiles that capture energy from pedestrian movement, potentially generating up to 5-8 watts per step.
Several pilot projects have demonstrated successful implementations, including a railway station in Paris that uses piezoelectric flooring to power LED lighting and information displays. The technology has also been incorporated into road surfaces, where passing vehicles generate electricity that can be stored or used immediately for street lighting and traffic signals.
The durability and longevity of modern piezoelectric materials have significantly improved, with new compounds showing minimal degradation over extended periods. This advancement, coupled with decreasing production costs, makes piezoelectric materials an increasingly viable option for energy-conscious building designs, particularly in high-footfall areas and infrastructure projects.
Smart Materials for Climate Adaptation
Phase Change Materials
Phase Change Materials (PCMs) represent a significant advancement in passive temperature regulation for buildings, offering an innovative solution to reduce energy consumption and enhance occupant comfort. These smart materials work by absorbing or releasing thermal energy during phase transitions, effectively stabilizing indoor temperatures without constant mechanical intervention.
The most commonly employed PCMs in construction are organic compounds, such as paraffin waxes, and inorganic materials like salt hydrates. When integrated into building elements such as walls, ceilings, or floors, these materials can absorb excess heat during peak daytime temperatures and release it when temperatures drop, creating a natural thermal buffer.
Recent developments have led to microencapsulated PCMs that can be incorporated into traditional building materials like concrete, gypsum board, and insulation. This integration allows for seamless implementation without compromising structural integrity or aesthetic requirements. Studies have shown that buildings utilizing PCM technology can reduce heating and cooling energy consumption by 20-30% compared to conventional structures.
Leading manufacturers are now producing PCM-enhanced construction materials with melting points specifically engineered for different climate zones and building applications. For example, office buildings in hot climates might utilize PCMs with higher melting points (25-28°C) to prevent overheating during business hours, while residential buildings might employ materials with lower transition temperatures (21-23°C) for overnight comfort.
The technology’s effectiveness has been demonstrated in several high-profile projects worldwide, including the Zero Energy Building at the National University of Singapore, where PCM-integrated walls contributed to a 50% reduction in cooling loads. As energy efficiency requirements become more stringent, PCMs are positioned to become a standard feature in sustainable building design.
Adaptive Façade Systems
Adaptive façade systems represent a significant leap forward in building envelope technology, utilizing smart materials that automatically respond to environmental conditions. These innovative systems incorporate dynamic elements such as electrochromic glass, thermochromic materials, and shape-memory alloys that transform building exteriors from passive barriers into active environmental mediators.
The most advanced adaptive façades employ multiple layers of responsive materials. Electrochromic glazing, for instance, changes its opacity in response to electrical signals triggered by environmental sensors, optimizing natural light penetration and heat gain. This technology can reduce HVAC energy consumption by up to 30% in commercial buildings.
Kinetic façade elements, powered by smart actuators and environmental sensors, automatically adjust their position based on sun angle, wind conditions, and temperature. These systems often incorporate photovoltaic cells and advanced insulation materials, creating a multi-functional envelope that generates energy while regulating interior comfort.
Recent developments include self-healing materials that can repair minor damage autonomously, and phase-change materials (PCMs) that absorb and release heat at specific temperature thresholds. These PCMs are particularly effective in maintaining stable indoor temperatures while reducing energy demands.
The integration of artificial intelligence and machine learning algorithms enables these systems to learn from usage patterns and weather data, continuously optimizing their performance. For example, the Al Bahar Towers in Abu Dhabi demonstrate how computerized geometric screens can automatically unfold and retract, reducing solar gain by up to 50%.
As climate challenges intensify, adaptive façade systems are becoming essential components of sustainable building design, offering unprecedented control over building performance while significantly reducing environmental impact.
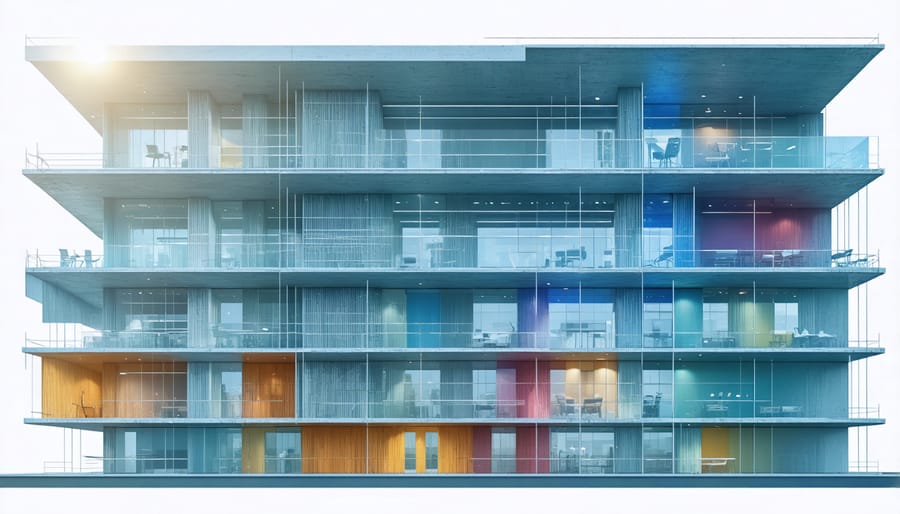
The evolution of building materials stands at a pivotal moment in construction history, where innovation meets necessity. As we’ve explored throughout this article, the future of construction materials promises to revolutionize how we build, maintain, and inhabit our structures. These advanced materials offer unprecedented combinations of durability, sustainability, and performance that were unimaginable just a decade ago.
The integration of self-healing concrete, transparent aluminum, and aerogels into mainstream construction practices signals a fundamental shift in building methodology. These materials not only address current challenges in construction but also anticipate future needs, particularly in energy efficiency and environmental impact reduction. Industry leaders estimate that implementing these innovative materials could reduce construction-related carbon emissions by up to 50% by 2040.
Perhaps most significantly, these materials represent more than just technological advancement – they embody a new philosophy in construction that prioritizes long-term sustainability without compromising structural integrity or economic viability. The successful adoption of these materials will require continued collaboration between researchers, manufacturers, and construction professionals, along with updated building codes and standards.
As we look ahead, it’s clear that these innovative materials will play a crucial role in shaping resilient, sustainable cities. The construction industry’s ability to adapt and embrace these technologies will determine not only the future of building practices but also our capacity to create structures that serve both humanity and the environment for generations to come.