Revolutionizing construction through automated additive manufacturing, 3D house printing robots are transforming how we build residential structures. These autonomous systems, emerging as frontrunners among modern building technologies, can now erect a complete house foundation and walls within 24-48 hours – a process that traditionally takes months using conventional methods.
By extruding specialized concrete mixtures layer by layer through computer-controlled nozzles, these sophisticated machines achieve unprecedented precision while reducing labor costs by up to 80% and construction waste by 60%. Industry leaders like ICON, COBOD, and Apis Cor have already deployed these systems in commercial projects across four continents, demonstrating their viability in real-world applications.
As construction firms face mounting pressure to address housing shortages, labor scarcity, and sustainability requirements, 3D printing robots offer a scalable solution that meets these challenges head-on. This technology not only accelerates construction timelines but also enables architectural designs previously impossible with traditional building methods, marking a paradigm shift in residential construction methodology.
The Technology Behind Construction 3D Printing Robots
Hardware Components and Design
3D house printing robots integrate sophisticated hardware components that work in harmony to enable precise construction operations. The primary component is a multi-axis robotic arm system, typically featuring 6-7 degrees of freedom, which provides the necessary range of motion and positioning accuracy for concrete extrusion. These arms are engineered to handle payloads of 100-250 kg, accommodating the substantial weight of construction materials.
The extrusion system consists of a specialized nozzle design that manages material flow and deposition. Modern systems utilize computer-controlled pumping mechanisms with pressure sensors to maintain consistent material viscosity and flow rates. The nozzle diameter typically ranges from 20-40mm, allowing for optimal layer height and width control during the printing process.
Mobility is achieved through either gantry-based systems or tracked platforms. Gantry systems offer superior stability and precision but are limited by fixed dimensions, while tracked platforms provide greater versatility for different construction sites. Advanced models incorporate stabilizing outriggers and auto-leveling systems to ensure precise operation on uneven terrain.
The control system integrates multiple sensors, including laser scanners and position encoders, to maintain spatial awareness and printing accuracy. These components are protected by ruggedized housings designed to withstand construction site conditions, including exposure to concrete splatter, dust, and varying weather conditions. Power management systems typically utilize industrial-grade batteries or direct power connections, depending on site requirements and operational duration needs.
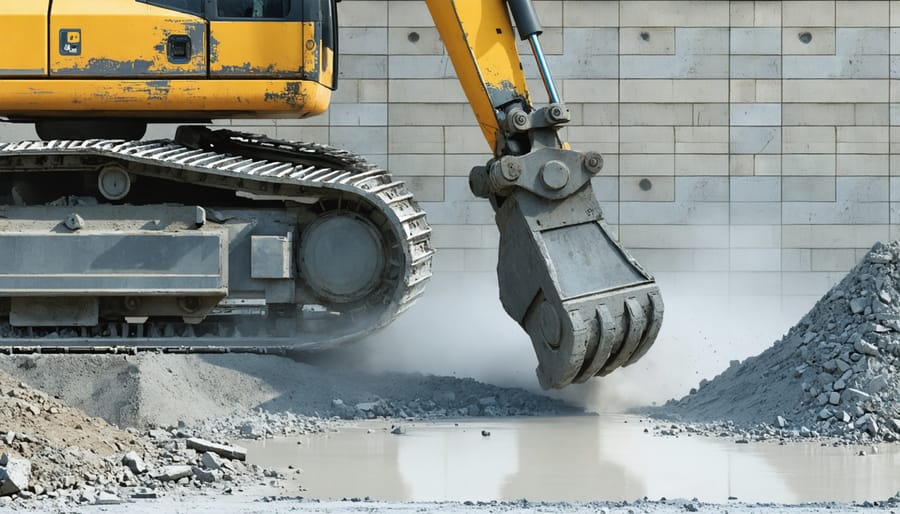
Material Science and Printing Materials
The success of 3D house printing heavily depends on the specialized concrete mixtures used in the process. These materials must meet specific requirements for flowability, setting time, and structural integrity. The most commonly used material is a proprietary cement-based composite that contains Portland cement, supplementary cementitious materials (SCMs), fine aggregates, and chemical admixtures.
Critical properties of the printing material include thixotropy – the ability to flow under pressure but maintain shape after extrusion – and controlled setting time to ensure proper layer adhesion. Modern formulations typically achieve compressive strengths ranging from 4,000 to 6,000 PSI, making them suitable for load-bearing applications.
Recent developments have introduced sustainable alternatives, including geopolymer concrete mixtures that reduce carbon emissions by up to 60% compared to traditional concrete. Some manufacturers have successfully incorporated recycled materials and industrial by-products, such as fly ash and silica fume, to enhance material properties while improving environmental performance.
The mix design must also account for local climate conditions, as temperature and humidity significantly impact material behavior during printing. Advanced additives are often incorporated to modify viscosity, control shrinkage, and enhance durability. Quality control systems continuously monitor material properties throughout the printing process, ensuring consistent performance and structural integrity of the final product.
Real-World Applications and Case Studies
Commercial Projects
Several construction technology companies have successfully deployed 3D house printing robots in large-scale commercial projects, demonstrating the technology’s viability beyond prototype demonstrations. Notable examples include the 2021 completion of a 5,000-square-foot commercial building in Dubai using COBOD’s BOD2 printer, and the construction of a multi-unit residential complex in Mexico by ICON Technology.
These commercial applications have revealed significant advantages in terms of construction speed and labor efficiency. Projects that traditionally required 6-8 months can now be completed in 2-3 months, with labor requirements reduced by up to 70%. Cost analysis from completed projects indicates potential savings of 20-40% compared to traditional construction methods, particularly in markets with high labor costs.
However, successful commercial implementation requires careful consideration of logistics and infrastructure. Projects need extensive site preparation, including level foundations and protected printing environments. The technology has proven most effective in regions with consistent weather conditions and where traditional construction faces significant challenges.
Recent commercial successes include multi-story apartment buildings in China, community housing projects in Africa, and industrial facilities in Europe. These projects have demonstrated various applications, from affordable housing developments to high-end architectural designs. The scalability of these operations has improved with each project, as teams develop more efficient workflows and overcome initial implementation challenges.
The most successful commercial applications have combined 3D printing with conventional construction methods, creating hybrid approaches that maximize the benefits of both technologies while maintaining quality and meeting building codes.
Residential Developments
The residential construction sector has witnessed significant advancement through the implementation of 3D printing robots, with several notable projects demonstrating the technology’s viability. In Austin, Texas, ICON completed a development of four 3D-printed homes in 2021, each constructed in under 48 hours, showcasing remarkable efficiency gains over traditional building methods. These homes featured 2,000-square-foot floor plans and met all local building codes while reducing construction waste by approximately 60%.
In the Netherlands, Project Milestone achieved a milestone with Europe’s first commercially rented 3D-printed house in Eindhoven. The boulder-shaped single-story home demonstrated the technology’s capability to create complex geometries while maintaining structural integrity. The project utilized a custom-designed concrete mixture that optimized both printability and durability.
Dubai’s ambitious 3D printing strategy has resulted in multiple residential developments, including a 6,900-square-foot two-story villa printed in 2023. The structure incorporated advanced thermal insulation properties and achieved a 50% reduction in labor costs compared to conventional construction methods.
In Mexico, the New Story charity partnership deployed 3D printing robots to construct affordable housing communities, completing 50 homes in Tabasco. Each 500-square-foot home was printed in 24 hours, demonstrating the technology’s potential for addressing housing shortages in developing regions.
These projects consistently report 20-40% cost savings, 30-60% faster construction times, and significant reductions in material waste. However, challenges remain in standardizing processes across different climate zones and ensuring long-term durability. Current developments focus on improving material formulations and expanding the range of architectural designs achievable through 3D printing technology.
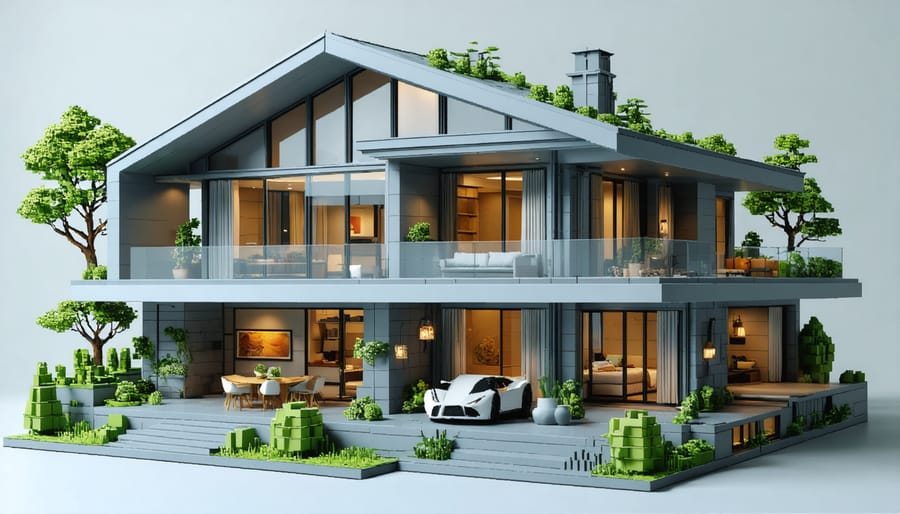
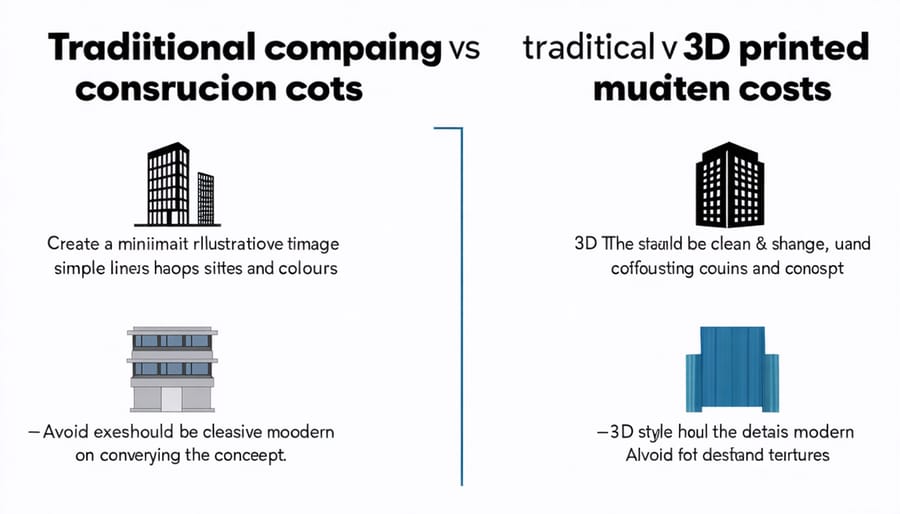
Cost-Benefit Analysis
The implementation of 3D house printing robots presents a compelling financial case when compared to traditional construction methods. Recent industry analysis reveals that these automated systems can reduce labor costs by 40-60% and cut overall construction expenses by 20-35% on typical residential projects. This significant reduction stems primarily from decreased labor requirements, minimal material waste, and shortened project timelines.
Initial investment in a 3D construction printer ranges from $150,000 to $1,000,000, depending on size and capabilities. While this represents a substantial upfront cost, ROI calculations indicate potential recovery within 2-3 years for companies maintaining steady project pipelines. With increased construction technology funding available, these initial costs are becoming more manageable for many firms.
Traditional construction methods typically require 6-8 months for a standard single-family home, while 3D printing can complete the basic structure in 1-2 weeks. This acceleration translates to reduced carrying costs, earlier occupancy, and faster project turnover. Material costs also favor 3D printing, with specialized concrete mixtures proving 30% more cost-effective than traditional building materials when factoring in reduced waste and transportation expenses.
Labor efficiency presents another significant advantage. A 3D printing system can operate with a crew of 2-3 trained technicians, compared to the 10-15 workers needed for conventional construction. This reduction not only decreases direct labor costs but also minimizes scheduling complexities and workplace safety risks.
However, considerations must include maintenance costs, specialized training requirements, and potential technological limitations. Current estimates suggest annual maintenance expenses of 5-7% of the initial investment, while operator training programs range from $5,000 to $15,000 per technician. These factors must be carefully weighed against the technology’s benefits when planning implementation strategies.
Regulatory Landscape and Industry Standards
The regulatory landscape for 3D printed construction continues to evolve as this innovative technology gains wider adoption. Currently, most jurisdictions are adapting existing building codes to accommodate 3D printed structures, while others are developing specific guidelines for this emerging construction method.
In the United States, the International Code Council (ICC) has begun incorporating provisions for 3D printed construction into building codes. The ICC-ES AC509 acceptance criteria, established in 2021, provides guidelines for evaluating 3D printed concrete walls as structural elements. Similarly, the American Concrete Institute (ACI) has formed Committee 564 to develop technical standards for 3D printed concrete construction.
European regulations are generally more advanced, with the European Committee for Standardization (CEN) working on harmonized standards for additive manufacturing in construction. Countries like Germany and the Netherlands have already implemented interim guidelines for 3D printed buildings, focusing on structural integrity, material performance, and safety requirements.
Key regulatory considerations include:
– Material certification requirements
– Structural performance validation
– Quality control protocols
– On-site safety procedures
– Environmental impact assessments
– Workforce certification standards
Industry stakeholders are collaborating with regulatory bodies to establish standardized testing methods and performance criteria. The UL 3401 standard, for instance, provides evaluation criteria for 3D printed building construction components. ASTM International’s Committee F42 on Additive Manufacturing Technologies is also developing relevant standards for construction applications.
Insurance providers and financial institutions are simultaneously developing risk assessment frameworks for 3D printed buildings, which is crucial for project financing and liability coverage. These frameworks consider factors such as durability, maintenance requirements, and long-term performance data.
As the technology matures, regulatory frameworks are expected to become more comprehensive and standardized across regions, facilitating wider adoption while ensuring safety and quality standards are maintained.
Future Implications for Construction Industry
The integration of 3D house printing robots is poised to revolutionize the construction industry over the next decade. Industry analysts project that by 2030, up to 25% of new residential construction projects could utilize this technology, fundamentally altering traditional building processes and workforce dynamics.
The most significant impact will likely be on labor markets, with a shift from traditional construction roles to more specialized technical positions. While some manual labor jobs may decrease, new opportunities will emerge in robot operation, maintenance, and digital construction planning. This transformation will require construction companies to invest in comprehensive training programs and digital skill development.
Construction methodologies will evolve toward more integrated digital workflows, combining Building Information Modeling (BIM) with automated construction processes. This integration, aligned with sustainable construction practices, will enable greater precision, reduced waste, and improved project timelines.
The industry’s supply chain will also undergo significant changes, with materials manufacturers developing specialized printing materials and construction companies adapting their procurement strategies. This evolution will likely lead to new industry standards and certifications specific to 3D-printed structures.
For construction businesses, early adoption of this technology could provide a competitive advantage, though careful consideration of implementation costs and regulatory compliance will be crucial. The success of this transition will depend on collaboration between technology providers, regulatory bodies, and construction professionals to establish robust frameworks for quality control and safety standards.
The emergence of 3D house printing robots represents a transformative shift in construction technology, promising to address critical industry challenges such as labor shortages, cost escalation, and project timelines. As demonstrated throughout this analysis, these systems offer significant advantages in speed, precision, and waste reduction while maintaining high construction quality standards. The technology continues to evolve rapidly, with improvements in materials science, printing techniques, and automation capabilities driving innovation forward.
Looking ahead, industry experts project widespread adoption of 3D construction printing within the next decade, particularly as regulatory frameworks mature and cost-effectiveness improves. The integration of artificial intelligence, advanced sensors, and improved material formulations will further enhance these systems’ capabilities. However, success will depend on continued collaboration between technology developers, construction professionals, and regulatory bodies to establish standards and best practices. For construction leaders considering implementation, now is the time to evaluate this technology’s potential impact on their operations and begin planning for its integration into their project delivery methods.