In an era of increasing grid vulnerabilities and rising energy costs, microgrid solutions have emerged as a transformative approach to power management in commercial buildings. These autonomous energy systems combine renewable sources, advanced storage capabilities, and intelligent distribution networks to deliver unprecedented control over energy consumption and reliability. By seamlessly integrating solar arrays, battery storage, and sophisticated control systems, microgrids enable facilities to operate independently from the main power grid during outages while optimizing energy costs during normal operations.
Recent data reveals that commercial microgrids can reduce energy expenses by 20-30% while providing critical resilience against power disruptions that cost U.S. businesses over $150 billion annually. Forward-thinking facility managers and building owners are increasingly recognizing microgrids as not merely a backup power solution, but as a strategic investment in operational efficiency, sustainability, and long-term cost control.
This comprehensive analysis explores the technical foundations, implementation strategies, and proven benefits of microgrid solutions for commercial buildings, offering decision-makers a roadmap to energy independence and operational excellence. From system architecture to ROI calculations, we’ll examine how leading organizations are leveraging this technology to future-proof their energy infrastructure.
The Building Energy Revolution: Understanding Microgrid Technology
Core Components of Building Microgrids
A successful microgrid implementation relies on three fundamental components working in harmony. First, diverse power generation sources form the foundation, typically combining renewable energy systems like solar panels and wind turbines with conventional backup generators. This multi-source approach ensures reliable power supply while maximizing sustainability.
The integration of advanced energy storage solutions represents the second critical component. Modern battery systems, such as lithium-ion arrays or flow batteries, enable microgrids to store excess power during peak generation periods and deploy it when needed, ensuring continuous operation during outages or high-demand periods.
The third essential component is the control and automation infrastructure, incorporating smart grid technology with sophisticated monitoring systems. This includes power management modules, distribution equipment, and intelligent controllers that optimize energy flow, manage load balancing, and enable seamless switching between grid-connected and island modes.
Additional components include protection devices, power quality equipment, and communication systems that ensure safe, efficient operation. The microgrid control system must also integrate with building management systems (BMS) to coordinate energy usage with HVAC, lighting, and other building systems, maximizing overall efficiency and reducing operational costs.
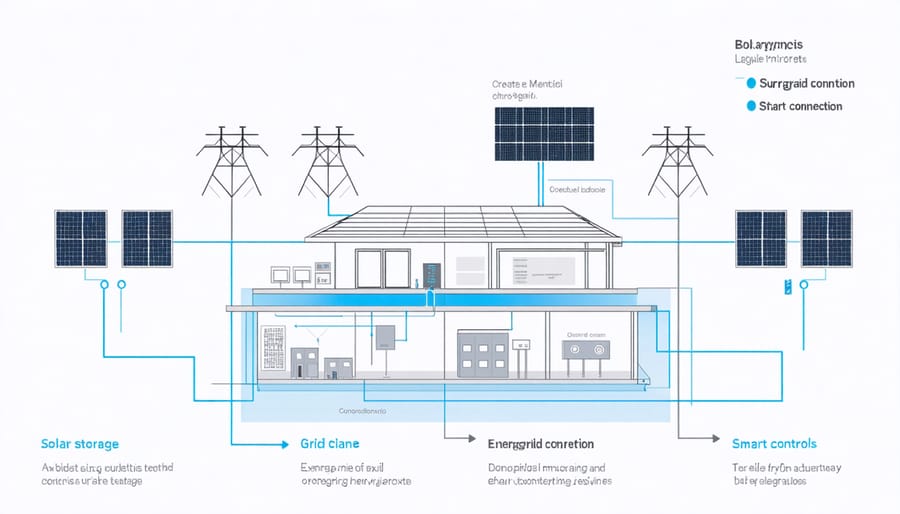
Integration with Existing Building Infrastructure
Integrating microgrid solutions with existing building infrastructure requires careful consideration of multiple technical interfaces and control systems. The process typically begins with a comprehensive assessment of the building’s electrical distribution system, including main switchgear, distribution panels, and emergency power systems.
Modern microgrids employ advanced control systems that seamlessly interface with Building Management Systems (BMS) through standardized protocols such as BACnet or Modbus. These systems coordinate power flow between traditional utility connections and microgrid resources, including on-site generation, energy storage, and renewable sources.
The integration process involves installing intelligent switchgear that can automatically isolate the building from the main grid during outages or economic optimization periods. Power quality monitoring equipment and advanced metering infrastructure (AMI) are deployed at key points to ensure system stability and enable real-time load management.
Critical considerations include maintaining proper voltage regulation, frequency synchronization, and phase alignment when transitioning between grid-connected and islanded operations. Modern microgrid controllers utilize sophisticated algorithms to manage these transitions while ensuring uninterrupted power supply to essential building systems.
Real-World Implementation Success Stories
Commercial Office Complex Case Study
The One Market Plaza in San Francisco serves as a prime example of successful microgrid implementation in a commercial office setting. This 1.6-million-square-foot complex, comprising two towers and an historic building, underwent a comprehensive microgrid retrofit in 2019 that has since delivered remarkable results.
The microgrid system includes a 500kW solar array installed across available roof space, a 2MWh battery storage system, and smart building controls integrated with the existing building management system. The implementation also features advanced load management capabilities and automated demand response protocols.
Key performance metrics demonstrate the project’s success: energy consumption reduced by 35% compared to pre-implementation levels, peak demand charges decreased by 42%, and an annual utility cost savings of $875,000. During a regional power outage in 2021, the microgrid maintained critical operations for 72 hours, ensuring business continuity for tenant operations.
The project’s $4.2 million investment achieved payback within 4.8 years through combined energy savings and demand response program revenues. Additionally, the building earned LEED Platinum certification, partly due to its innovative energy infrastructure.
This implementation showcases how modern microgrids can enhance building resilience while delivering substantial operational cost savings and supporting sustainability goals in large commercial complexes.
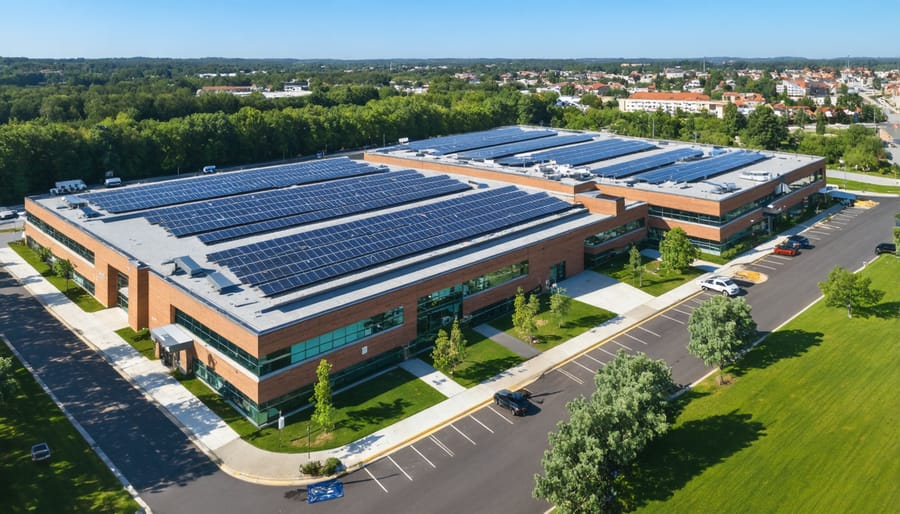
Healthcare Facility Energy Independence
Healthcare facilities require uninterrupted power supply to maintain critical operations and ensure patient safety. Microgrids offer these institutions a reliable solution for energy independence while reducing operational costs and environmental impact. Recent implementations have demonstrated that healthcare facilities can maintain full functionality during grid outages, potentially saving lives during natural disasters or infrastructure failures.
A notable example is the microgrid system at Montefiore Medical Center in New York, which combines CHP (Combined Heat and Power) technology with sophisticated control systems to ensure continuous power delivery. This installation not only provides emergency backup but also optimizes daily energy consumption, resulting in approximately 30% reduction in energy costs.
Critical components of healthcare facility microgrids typically include:
– Redundant power generation systems
– Advanced battery storage solutions
– Intelligent load management systems
– Automated transfer switches
– Real-time monitoring and control interfaces
Implementation challenges often center around integrating these systems with existing medical equipment and meeting stringent regulatory requirements. However, the benefits far outweigh the initial complexities. Healthcare facilities with microgrids report enhanced operational reliability, improved power quality for sensitive medical equipment, and significant cost savings through peak load management.
ROI analysis from multiple implementations shows typical payback periods of 5-7 years, with additional value derived from increased resilience and reduced insurance premiums. This makes microgrids an increasingly attractive solution for healthcare facility managers seeking both operational security and financial efficiency.
Financial Benefits and ROI Analysis
The financial benefits of implementing microgrid solutions extend far beyond simple utility cost reduction, offering a compelling return on investment (ROI) for commercial building owners and operators. When integrated with comprehensive building energy management strategies, microgrids typically deliver ROI within 5-7 years, with some systems achieving payback in as little as 3 years.
Recent case studies demonstrate that commercial buildings implementing microgrid solutions achieve average energy cost savings of 25-35% annually. These savings come from multiple sources: reduced grid electricity consumption, demand charge avoidance, and potential revenue from participating in demand response programs. For a typical 100,000-square-foot commercial building, this can translate to annual savings of $100,000-150,000.
Initial investment costs vary significantly based on system size and complexity:
– Basic microgrid systems: $1-2 million per MW of capacity
– Advanced systems with storage: $2-4 million per MW
– Complex systems with multiple generation sources: $4-6 million per MW
However, various financial incentives can substantially reduce these costs:
– Federal tax credits (up to 30% of system cost)
– State-level grants and rebates
– Utility incentive programs
– Performance-based incentives
The long-term value proposition extends beyond direct energy savings. Microgrids provide:
– Enhanced property value (typically 5-10% increase)
– Reduced maintenance costs through predictive management
– Revenue generation through grid services
– Business continuity benefits during outages
– Carbon reduction credits and environmental compliance value
When calculating ROI, organizations should consider both quantifiable benefits (energy savings, demand charge reduction) and qualitative advantages (reliability, sustainability credentials). Most commercial installations achieve internal rates of return between 12-18%, making microgrids an increasingly attractive investment for forward-thinking building owners.
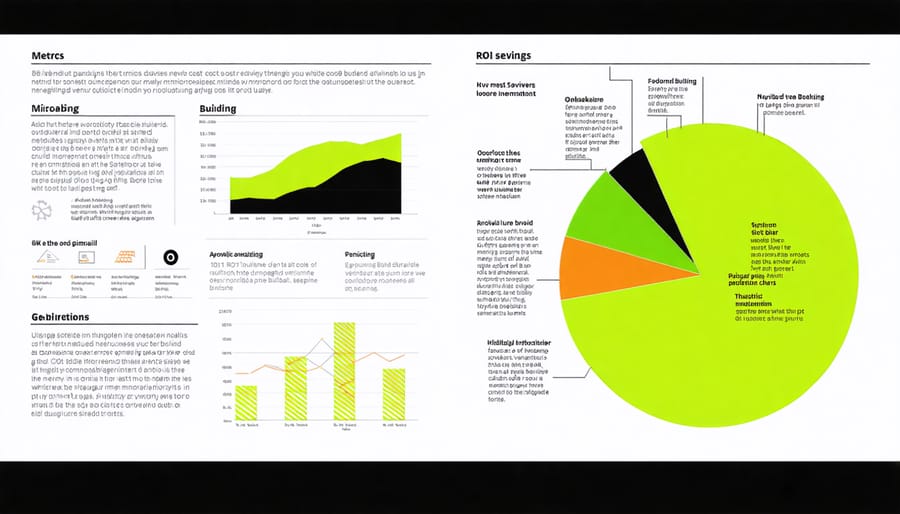
Regulatory Compliance and Future Trends
Current Regulatory Framework
The regulatory landscape for microgrid implementation varies significantly across jurisdictions, with federal, state, and local requirements often intersecting. At the federal level, the Federal Energy Regulatory Commission (FERC) oversees interstate electricity transmission and wholesale power sales, while state public utility commissions regulate distribution-level interconnection standards and retail electricity sales.
Key compliance requirements include IEEE 1547 standards for interconnection, which ensure safe integration with the main grid, and UL 1741 certification for inverters and power conversion equipment. Building codes and electrical standards, such as NFPA 70 (National Electrical Code), must also be strictly followed during microgrid installation.
Several states have enacted specific microgrid-enabling legislation, with California, New York, and Massachusetts leading the way through programs like California’s Electric Rule 21 and New York’s Reforming the Energy Vision (REV) initiative. These frameworks provide clear guidelines for permitting, interconnection procedures, and operational requirements.
Project developers must also consider environmental regulations, particularly for systems incorporating generation assets, and coordinate with local utilities regarding interconnection agreements and operating procedures.
Emerging Technologies and Future Applications
The future of microgrid solutions is rapidly evolving with groundbreaking technological advancements. Artificial Intelligence (AI) and machine learning algorithms are revolutionizing how decentralized energy systems operate, enabling predictive maintenance and automated load balancing with unprecedented accuracy.
Blockchain technology is emerging as a game-changer for peer-to-peer energy trading within microgrids, allowing building owners to securely buy and sell excess power. Advanced energy storage solutions, including solid-state batteries and hydrogen fuel cells, are set to dramatically improve storage capacity and efficiency.
Internet of Things (IoT) integration is becoming more sophisticated, with smart sensors and controllers enabling real-time optimization of energy distribution. These developments are complemented by advanced weather forecasting systems that help maximize renewable energy utilization.
Industry experts predict that building-integrated photovoltaics (BIPV) will become standard in new construction, seamlessly incorporating solar generation into building materials. Additionally, vehicle-to-grid (V2G) technology will transform electric vehicle fleets into mobile power storage units, adding another layer of flexibility to microgrid operations.
Microgrid solutions represent a transformative approach to building energy management, offering compelling benefits that extend beyond mere cost savings. By implementing these systems, building owners and operators can achieve enhanced energy reliability, reduced carbon emissions, and improved operational resilience. The integration of renewable energy sources, advanced storage systems, and intelligent control mechanisms creates a robust framework for sustainable building operations.
To successfully implement a microgrid solution, organizations should begin with a comprehensive energy audit and needs assessment. This should be followed by developing a detailed implementation strategy that includes selecting appropriate technology partners, securing necessary permits, and establishing clear performance metrics. Key considerations include scalability, maintenance requirements, and integration with existing building management systems.
For optimal results, organizations should adopt a phased approach to implementation, starting with pilot projects in critical areas before expanding system-wide. Regular monitoring and optimization of the microgrid system ensure maximum efficiency and return on investment. By partnering with experienced providers and leveraging available incentives and grants, organizations can significantly reduce initial implementation costs while maximizing long-term benefits.
The future of building energy management lies in microgrid solutions, making them an essential consideration for forward-thinking construction professionals and facility managers.